Femoral fractures occur commonly in both cats and dogs accounting for 20–26% of all fractures and 45% of all long bone fractures (Whitehair and Vasseur, 1992). They appear to occur more commonly in younger animals, with one study reporting that 69% of femoral fractures occurred at or below 2 years of age. These fractures usually occur secondary to high impact injuries from vehicular trauma, falls or gunshot wounds (Whitehair and Vasseur, 1992). Fractures that result from minimal trauma warrant diagnostics to rule out an underlying pathological condition, most commonly neoplasia (Beale, 2004). Because of the extensive overlying musculature, which both protects the skin from the sharp bone fragments and acts as a shock absorber during trauma, the majority of femoral fractures remain closed (Braden et al, 1995; Beale, 2004). Only 8% of femoral fractures are reported to be open, which is substantially lower than corresponding percentages for the distal tibia where rates of up to 20% have been reported (Hayashi and Kapatkin, 2012).
Imaging
Radiographic assessment should include, as a minimum, mediolateral and craniocaudal views of both femurs. The craniocaudal view can be obtained either by extending the limb with the animal in dorsal recumbency or by using a horizontal beam. If the option with the animal in dorsal recumbency is selected, the surgeon should be aware that as the femur cannot be positioned parallel to the cassette, distortion will occur which may alter the perception of fracture lines and affect templating for implants (Guiot et al, 2012). Oblique views of the femur are of limited diagnostic value, but their use for the distal femur in combination with tangential views of the trochlea may be of assistance, particularly when unicondylar fractures are encountered, as these are oft en minimally displaced (McLaughlin, 1993). The use of stress radiographs in varus or valgus may also assist with identification of minimally displaced condylar fractures.
If fractures of the proximal femur, affecting the epiphysis, femoral capital physis or subtrochanteric area, are suspected or identified then ventrodorsal views of the pelvis are also recommended (Rendano et al, 1980) both with the pelvic limbs extended and in a ventrodorsal frog-leg view. The ventrodorsal view with the pelvic limbs extended results in hip extension and resultant tightening of the joint capsule, which may reduce capital physeal fractures, rendering them more difficult to assess. The fracture is more likely to displace on the frog-leg view, making it more easily identifiable (Figure 1). This frog-leg view may also facilitate the identification of sclerosis and osteolysis which may be seen in cases with more chronic fractures (Craig, 2001), which may subsequently affect therapeutic decision-making.
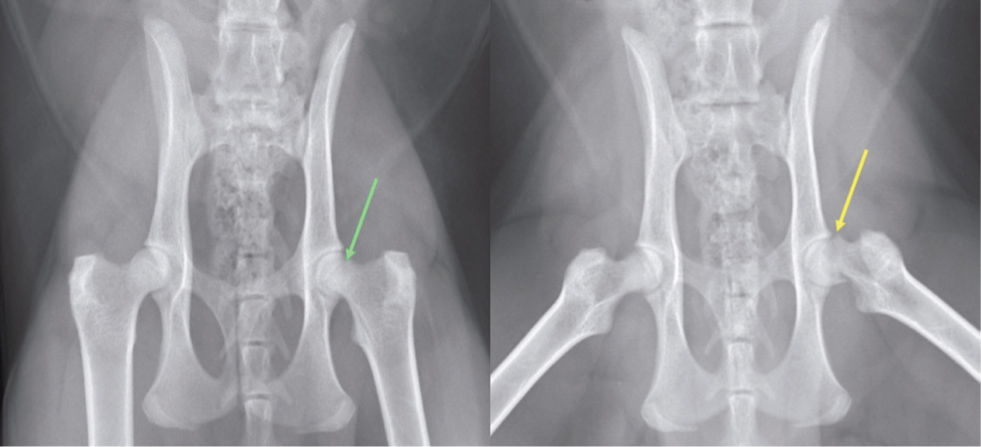
While oblique and stress views can certainly provide supplementary information to conventionally positioned radiographs, if complex fractures are encountered, the use of computed tomography (CT) should be considered. Imaging using CT is a valuable diagnostic tool for assessment of the proximal and distal femur particularly in cases of comminution, articular involvement or where fissures projecting proximally or distally are suspected. This imaging modality allows complete evaluation of the bone with multiple image planes being obtained (Figure 2) and provides a more thorough assessment of joint injury than is possible with radiography. While magnetic resonance imaging (MRI) has become the diagnostic imaging method of choice in humans (Reichter et al, 1987) as it provides valuable information regarding soft tissue and cartilage, the associated cost and the requirement for general anaesthesia means it is not commonly used in small animal patients.

Fracture classification
Femoral fractures can be classified based on their anatomic location (Daly, 1978; Braden et al, 1995; Beale, 2004), including:
- Fractures of the proximal epiphysis
- Proximal physeal fractures
- Subcapital fractures
- Fractures of the femoral neck
- Trochanteric fractures
- Subtrochanteric fractures
- Fractures of the proximal, middle or distal shaft
- Supracondylar fractures
- Distal physeal fractures
- Unicondylar fractures
- Bicondylar fractures
- Fractures affecting the femoral trochlea.
In addition, physeal fractures are classified according to the Salter–Harris classification (Salter and Harris, 1963). Fractures of the femoral shaft are encountered most commonly, representing over 50% of femoral fractures, with distal and proximal physeal fractures being the next most common anatomic locations (Braden et al, 1995).
Fractures of the proximal femur are also often classified as intracapsular or extracapsular, based upon their location with respect to the joint capsule (Daly, 1978). From proximal to distal, intracapsular fractures include epiphyseal, physeal, subcapital and femoral neck fractures. Similarly, from proximal to distal, extracapsular fractures include basilar neck, intertrochanteric and subtrochanteric fractures. This classification system was proposed because of the joint capsule's intricate association with the vascular supply to the femoral head and neck (Daly, 1978). It was speculated that intracapsular fractures would be more likely to be associated with avascular necrosis and that this classification system could be used as a prognostic indicator (Daly, 1978).
Femoral fractures vary greatly in their complexity but can also be classified by their configuration. Most fractures are reported to be simple, with just two segments approximately 50% of the time (Braden et al, 1995). However, one third of fractures are severely comminuted with four or more fragments (Braden et al, 1995). All fracture types described for long bones including transverse, short or long oblique, spiral, butterfly, segmental and comminuted, may be encountered (Braden et al, 1995; Beale, 2004; Piermattei et al, 2006).
Treatment
In general, femoral fractures are not amenable to treatment with external coaptation because of the anatomy of the femur and its surrounding soft tissue envelope (Beale, 2004). Therefore, surgical stabilisation or a salvage procedure will be required. Careful preoperative assessment and planning of treatment is mandatory if optimal results are to be achieved. Sufficient imaging should be performed to allow fracture classification as detailed above, including careful assessment for articular involvement. When selecting an implant system, a thorough understanding of the forces to which the implants will be subjected is essential (Autefage, 2000; Stiffler, 2004).
Selecting an approach
One of the first decisions to make involves the surgical approach. Femoral fractures can be treated using the principles of biological osteosynthesis, with minimally invasive ‘look-but-do-not-touch’ approaches, or by anatomic reduction and stabilisation. The surgical approach will vary depending upon whether the fracture is to be anatomically reconstructed and which stabilisation technique is selected (Beale, 2004).
When fractures are amenable to closed reduction and stabilisation using minimally invasive techniques, this likely reduces injury to the fracture haematoma, the vascular supply and, in juxta-articular fractures, the articular cartilage (Guiot et al, 2012). This approach expedites healing and is associated with low patient morbidity if performed appropriately (Beale, 2004). In minimally invasive osteosynthesis, alignment of bone fragments is achieved with indirect reduction techniques such as fracture reduction under the plate, intra-operative skeletal traction using dedicated tables, linear or circular external skeletal fixators, fracture distraction, limb hanging, intramedullary pinning, interlocking nailing, three-dimensional printed alignment constructs or a combination of these techniques (Peirone et al, 2012; Lynch and Davies, 2019; Dejardin et al, 2020). A recent study showed that postoperative alignment after fracture reduction under the plate, combined with minimally invasive plate osteosynthesis for non-articular fractures of the femur, led to clinically acceptable results regarding postoperative limb alignment without the use of intraoperative fluoroscopy (Cabassu, 2019). It should be recognised that the use of minimally invasive techniques does render achieving appropriate limb alignment more difficult because the fracture site is more difficult to visualise. In the aforementioned study comprehensive preoperative planning, combined with precise precontouring of the plate to the bone, was instrumental in achieving these results (Cabassu, 2019).
While the popularity of minimally invasive approaches is increasing, open reductions and stabilisations of femoral fractures are more commonly performed. In order to retain a good prognosis basic principles must be followed, including using the appropriate surgical approach to preserve regional soft tissues and their attachments to bone fragments (Beale, 2004). If an open approach is selected for femoral shaft fractures, a lateral approach to the femur is generally appropriate for reduction and stabilisation of all fracture types (Brinker, 1957; Piermattei and Johnson, 2004).
For fractures involving the proximal femur and hip joint, several approaches have been described (Archibald et al, 1953; Brown, 1953; Hohn, 1965; Brown and Rosen, 1971; Wadsworth and Henry, 1974; Slocum and Hohn, 1975; Slocum and Devine, 1986; Piermattei and Johnson, 2004). The craniolateral approach to the hip provides adequate exposure of the proximal epiphysis, physis and neck and can be used for the majority of proximal fractures. However, in select cases where additional exposure is required, this may be achieved using the dorsal approach via osteotomy of the greater trochanter, or, in very young animals, the dorsal approach via tenotomy of the gluteal muscles (Piermattei and Johnson, 2004). A ventral approach has also been described, with the postulated advantage of not interfering with epiphyseal blood supply (Hohn, 1965; Slocum and Devine, 1986; L'Eplattenier and Montavon, 1997; Guerrero et al, 2005).
Multiple surgical approaches have been described for the distal femur, which can be used either individually or in combination (Paatsama, 1952; Piermattei and Johnson, 2004). Lateral, medial or combined approaches can be used, all of which are associated with parapatellar arthrotomy. A lateral approach is often preferred as it can easily be combined with the lateral approach to the femur for more proximal exposure (Piermattei and Johnson, 2004). Osteotomy of the tibial tuberosity has been suggested for additional exposure in cases of articular fracture. However, the morbidity associated with this approach typically negates any benefit gained from additional exposure (Piermattei and Johnson, 2004), therefore its use should be limited to severely comminuted articular fractures where acceptable visualisation cannot be achieved through less invasive approaches.
Proximal epiphyseal fractures
Fractures of the proximal epiphysis are rare, most commonly occurring in combination with coxofemoral luxation where the avulsed epiphyseal fragment often remains attached to the ligament of the femoral head (L'Eplattenier and Montavon, 1997; Vernon and Olmstead, 1983; Simpson and Lewis, 2002).
Therapeutic choice will depend on the size and number of epiphyseal fragments. The greater the number of fragments, and the smaller their size, the less likely it becomes that accurate anatomic reconstruction will be achieved. In most situations, satisfactory exposure will be achieved via a craniolateral approach to the hip (Piermattei and Johnson, 2004). Smaller fragments not amenable to fixation may be excised to prevent ongoing damage to the articular cartilage, but when the fragment is large enough anatomic reduction and rigid fixation are recommended. Following anatomic reduction, stabilisation can be achieved using Kirshner (K)-wires, small-diameter lag screws, or both depending on the size of the fragment. Ideally, a minimum of two implants should be used to counteract rotational forces and all implants should be countersunk beneath the articular surface (Tillson et al, 1994; Kuzma et al, 1989; Guerrero et al, 2005). Careful closure of the joint capsule is important to prevent postoperative coxofemoral luxation. Augmentation of the joint capsule may be considered in cases where the capsule is too severely damaged to allow a robust repair (Allen and Chambers, 1986; Braden and Johnson, 1988).
Femoral capital physeal fractures
Capital physeal fractures occur in skeletally immature animals, secondary to trauma (Simpson and Lewis, 2002). Femoral capital physeal fractures are also common in young adult cats, where they are often atraumatic, and are associated with capital physeal dysplasia (Lee, 1976; Culvenor et al, 1996; Forrest et al, 1999; Craig, 2001). Multiple risk factors for spontaneous femoral capital physeal fractures in cats over one year of age have been identified, including sex, reproductive status, delayed physeal closure and abnormally high body weight, with the condition being reported to be bilateral in 19-24% of cases (Walter et al, 2002; Harasen, 2004). Atraumatic fractures have also been reported sporadically in dogs, although this is significantly less common (Figure 3).
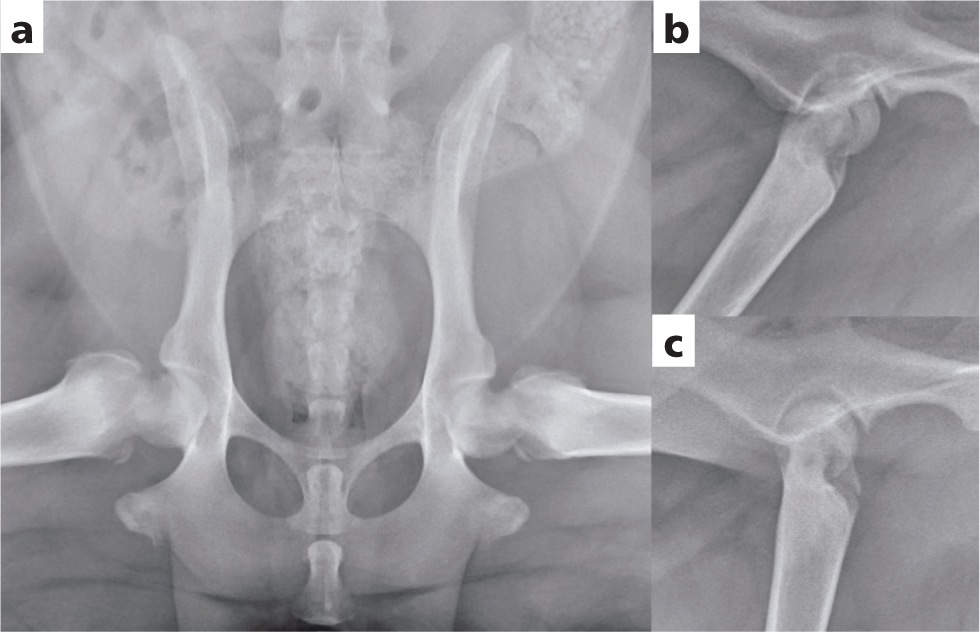
Regardless of whether fractures are traumatic or atraumatic, Salter-Harris type I fractures are by far the most commonly encountered type (Marretta and Schrader, 1983; DeCamp et al, 1989). In traumatic cases, concurrent separation of the trochanteric physis is noted in 11–15% of cases (Brinker, 1965; Denny, 1971; Gibson et al, 1991).
Reported treatment options for femoral capital physeal fractures include rest and non-surgical management, stabilisation with multiple pins or a lag screw, femoral head and neck excision, and total hip replacement (Daly, 1978; Kalis et al, 2012). While non-surgical treatment has been reported (Ordeberg et al, 1984), continued displacement of the capital epiphysis may occur and the displacement may progress to the formation of a pseudoarthrosis (Carney et al, 1991). Results of one study by Perez-Aparicio and Fjeld (1993), indicated that cats with femoral capital physeal fractures treated with rest and medical management developed a malunion or non-union causing persistent lameness. As such, in more recent literature, regardless of whether these fractures are acute or chronic, some form of surgical management is generally recommended (Kalis et al, 2012) (Figure 4). Results of previous reports (Daly, 1978; Culvenor et al, 1996; Fischer et al, 2004), support internal fixation as the treatment of choice for femoral capital physeal fractures where this is possible. Reconstruction of the fracture site is optimal for maintaining the hip joint and returning to normal anatomic configuration, which is believed to result in the fastest recovery and best function postoperatively (Culvenor et al, 1996).
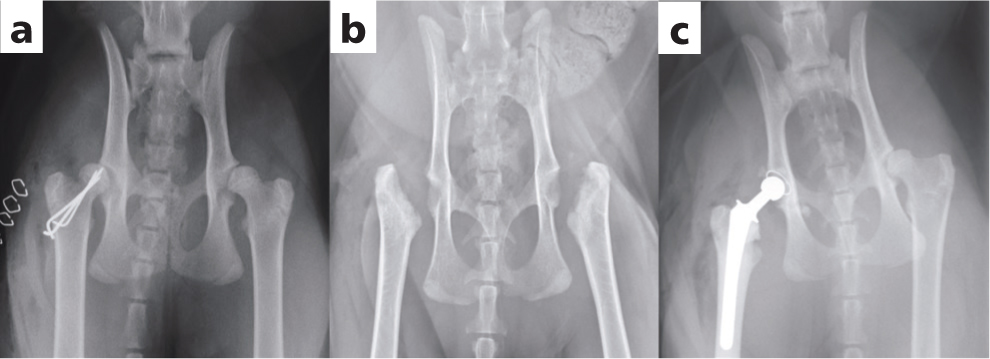
Femoral capital physeal fractures may be stabilised using open approaches or via closed reduction with intraoperative fluoroscopic guidance (Guiot et al, 2012; Boekhout-Ta et al, 2017). Fluoroscopic-assisted percutaneous pinning should generally be reserved for acute fractures with minimal displacement.
If an open approach is selected, the craniolateral approach to the hip is expected to provide satisfactory exposure (Piermattei and Johnson, 2004). The physis should be anatomically reduced. The L-shaped profile of the capital physis helps guide this reduction by providing landmarks for restoration of normal anatomy; it also offers some intrinsic stability that counteracts shear forces once reduction is achieved (Simpson and Lewis, 2002). Stabilisation is then achieved using either parallel or divergent K-wires, or in larger dogs, a lag screw. K-wires can be placed in either a normograde or retrograde fashion. Normograde insertion from distal to proximal is the least invasive option, although accurate pin placement can be somewhat challenging. Pin insertion should start on the lateral aspect of the femur, caudodistal to the greater trochanter at the level of the third trochanter. For optimal anchorage, the pin is angled so that its exit point is near the centre of the capital physis. Two or three pins are all placed where their trocar tips are just visible at the fracture site. The fracture is then reduced and once anatomic reduction has been achieved, the pins are driven across into the epiphyseal segment achieving maximal purchase of the femoral head without penetrating the articular surface.
Following primary repair, lameness significantly improves during a 4 week post-operative period. The placement of K-wires that results in anatomic, or near-anatomic reduction is likely to result in a good prognosis (Fischer et al, 2004). Despite the fact that the entire femoral capital epiphysis is intra-articular and therefore dependent on the ascending arteries of the femoral neck, few cases of avascular necrosis are reported (Craig, 2001). However, careful post-operative monitoring is recommended to ensure adequate progression of the osseous union. During healing, bone tends to be laid down on medial aspect of the neck of the femur, in agreement with Wolff's law, as the bone is being laid down in areas of increased stress (Fischer et al, 2004).
While primary repair of capital physeal fractures is considered ideal, chronic fractures with subsequent remodelling may impede accurate reduction and repair. In such cases, femoral head and neck excision or total hip replacement may be indicated (Kalis et al, 2012). Total hip replacement has been reported as a treatment option for capital physeal fracture in cats, with excellent results (Kalis et al, 2012).
Femoral neck fractures
Femoral neck fractures occur in dogs and cats of all ages after substantial trauma, but are most commonly seen in animals aged under 12 months (Daly, 1978; Papmahl Hollenberg, 1980; Stigen, 1986; Perez Aparicio and Fjeld, 1993). Surgical treatment is required for femoral neck fractures as conservative treatment generally leads to the formation of a hypertrophic pseudoarthrosis, with subsequent reductions in range of motion and persistent lameness (Papmahl Hollenberg, 1980; Perez Aparicio and Fjeld, 1993).
Reduction of the fracture and stabilisation with divergent K-wires or a lag screw is considered the optimal treatment. The use of a lag screw has been shown to be significantly stronger and also provides more interfragmentary compression (Lambrechts et al, 1993), which may be particularly beneficial in larger dogs. Femoral neck fractures are significantly more difficult to treat than capital physeal fractures, as they lack the inherent stability of the L-shaped physis and are exposed to considerable bending moments. The steeper the fracture line is relative to the long axis of the femoral neck, the greater the shear stresses at the fracture site and the higher the risk of non-union (Brinker, 1966; Hulse et al, 1974). Additionally, femoral neck fractures may be found in combination with other fractures including subtrochanteric femoral fractures, or fractures extending up through the femoral head (Beale, 2004).
If anatomic reduction and stabilisation is elected, both open and minimally invasive approaches can be appropriate (Figure 5). If an open approach is elected, a craniolateral approach to the hip combined with a lateral approach to the proximal femur is most commonly used, although dorsal approaches are also possible. Similarly to femoral capital physeal fractures, if K-wires are used they may be placed either normograde or retrograde. Optimal wire diameter will depend upon animal size, with a range between 0.7 mm to 1.6 mm being appropriate for most cats and small-to medium-breed dogs. If anatomic reduction and stabilisation is deemed unfeasible, femoral head and neck excision or total hip replacement remain as salvage treatment options.
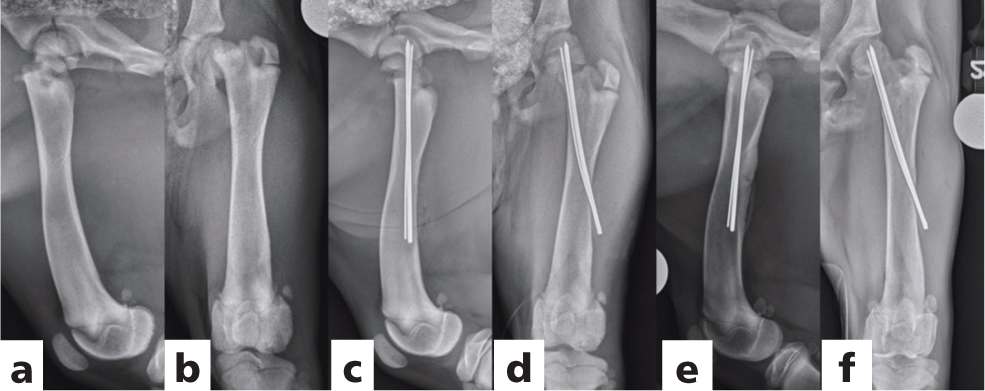
Trochanteric fractures
Fractures of the greater trochanter occur as avulsion fractures in immature animals (Denny, 1971; DeCamp et al, 1989; Prieur, 1989; Gibson et al, 1991). While they are frequently seen with concurrent ipsilateral fractures of the capital physis or femoral neck (Denny, 1971; DeCamp et al, 1989; Prieur, 1989; Gibson et al, 1991), they do occasionally occur as isolated fractures or in combination with other fractures of the femur or pelvis. Typically, the proximal fragment displaces cranially and proximally because of the pull of the attached middle gluteal muscle. These fractures should therefore be stabilised using a technique that will withstand the distractive gluteal force during weightbearing.
Open reduction and stabilisation results in a good functional outcome and is the standard treatment of choice for these fractures, as it is most likely to restore normal hip joint biomechanics. A craniolateral approach to the hip generally provides sufficient exposure. Potential stabilisation methods include a pin and tension band wire or, less commonly, a lag screw. If using a pin and tension band, two K-wires are directed across the greater trochanter from lateral to medial, perpendicular to the physis and parallel to each other, exiting the medial cortex of the proximal femur. A figure-of-eight tension band is then placed around the pins proximally and through a bone tunnel drilled from lateral to medial distally (Beale, 2004).
While surgical stabilisation is generally recommended, non-surgical management can occasionally be considered depending on the severity of displacement. If this is minimal, strict cage rest for 3–4 weeks may be sufficient to permit acceptable healing without loss of function (Beale, 2004). However, if displacement is significant, conservative treatment is not recommended as this results in malunion and altered development of the proximal femur resulting in loss of function (Beale, 2004).
Subtrochanteric fractures
Subtrochanteric fractures are relatively uncommon. They involve the proximal metaphyseal region of the femur distal to the trochanter (Simpson and Lewis, 2002) and may occur as isolated fractures, or in combination with femoral neck or shaft fractures. Subtrochanteric fractures are often highly comminuted and can be difficult to anatomically reduce and stabilise. Regardless of the complexity, surgical stabilisation is recommended.
Minimally invasive techniques are not commonly used for treatment of subtrochanteric fractures, as the inherently unstable nature of these fractures renders them difficult. The fracture is more commonly approached through a combined craniolateral approach to the hip and lateral approach to the diaphysis. While minimally invasive approaches may not be feasible, a biological approach should still be adopted with minimal dissection being performed as preserving the regional blood supply of the fracture fragments is critical for early bone healing (Guiot et al, 2012).
Subtrochanteric fractures may be anatomically reconstructed and stabilised or indirectly reduced and treated by biological osteosynthesis. Commonly used implant systems include bone plates, interlocking nails and plate-rod fixation as primary implants; with lag screws, K-wires and cerclage wires being used as secondary implants as required. Bone plating is typically the most effective method of fixation as there is often a paucity of bone stock proximally. The ability to contour the plate up and over the lateral aspect of the greater trochanter allows that limited bone purchase to be optimised. The use of interlocking nails in subtrochanteric fractures is limited to fractures where proximal bone stock is sufficient to allow placement of the proximal locking bolts. External fixators can also be applied adjunctively, but their use in the femur is less desirable.
Fractures of the femoral shaft
Several techniques have been described for stabilisation of femoral fractures, including intramedullary pins (with and without cerclage wires), external skeletal fixators, bone plates with screws, interlocking nails and combinations of these techniques.
Pins and wires
Although early reports of femoral fracture fixation describe the use of a single intramedullary pin, subsequent literature detailed an unacceptably high complication rate associated with this technique and it is now rarely recommended. While Denny (1991) reported that most femoral shaft fractures could be successfully managed in the cat using an intramedullary pin with or without cerclage wires, there are significant biomechanical limitations to these fixation devices. Femoral shaft fractures are subject to torsional shear, compression and bending forces and the intramedullary pin only gives good stabilisation against angular deformity. The inability of the intramedullary pin to counteract all forces at the fracture gap, and specifically to provide adequate axial and rotational stability, accounts for the high complication rates reported. Stack pinning does not significantly increase the resistance to rotational stability, when compared to a single intramedullary pin, and stack pinning for long bone fractures has been associated with a complication rate of greater than 50% (Gibson and Van Ee, 1991), so is also not recommended.
Intramedullary pin and cerclage wire fixation is only effective for stabilisation of long oblique or spiral fractures, and reconstructable butterfly fractures of the femoral shaft. Anatomic reconstruction is a prerequisite for this technique, as following this the intramedullary pin will resist bending forces and the cerclage wires will counteract the rotational and compressive forces. Less commonly used options to counteract the rotational and compressive forces, in conjunction with an intramedullary pin, include the use of lag screws or an adjunctive external skeletal fixator (Beale, 2004).
In order to satisfactorily resist bending forces, an intramedullary pin with a diameter approximating the diameter of the femoral isthmus should be chosen. Intramedullary pins can be placed either normograde or retrograde, but normograde placement is strongly recommended as it allows appropriate placement within the trochanteric fossa and medullary canal. Normograde placement also helps avoid impingement of the sciatic nerve (Fanton et al, 1983), minimises manipulation at the fracture site and helps maintain the fracture haematoma, encouraging healing. In order to further minimise the risk of sciatic nerve impingement, the hip should be held adducted and in maximal extension while the pin is inserted (Fanton et al, 1983; Palmer et al, 1988; Simpson and Lewis, 2002). The distal portion of the pin should be firmly embedded in the metaphyseal bone. Once placement is finalised, the pin should be cut and countersunk so that it is level with, or distal to, the top of the greater trochanter (Beale, 2004).
If cerclage wires are used to control rotational and compressive forces, these should be adequately sized to provide rotational and axial stability. In medium and large-breed dogs, 18 gauge wire is generally appropriate. Double-loop cerclage should be used with the wires applied very tightly (Roe, 1997; 2002). Loose wires lead to delayed healing by disruption of the blood supply, so must be avoided.
External skeletal fixators
External skeletal fixators are often used for biologic management of fractures, because of the lower implant-associated costs and the relative ease of application. They can also be used following either open or closed reduction of the fracture and, when applied appropriately, the vascular supply of the fracture fragments can be preserved very efficiently. However, external fixators are not commonly used as the sole method of repair in femoral fractures on account of the inherent mechanical and biological limitations of their use in this area.
Ideally, when applying an external skeletal fixator, the pins should be placed through safe corridors. A safe corridor is defined as a site where the pin may be placed from skin to bone without penetrating any neurovascular bundles or musculotendinous units (Marti and Miller, 1994). The use of external skeletal fixators in the femur has been discouraged as the bone has no real safe corridors (Marti and Miller, 1994). In prospective and retrospective case series involving external skeletal fixator use for stabilising femoral fractures, some form of pin tract inflammation or discharge was reported in approximately 60–80% of dogs and in 22% of cats (Peirone et al, 2002; Kirkby et al, 2008). If external fixators are used, owners should be informed that postoperative care is more demanding, with regular bandage changes and pin tract management being anticipated (Beale, 2004).
Additionally, when considering the use of femoral external skeletal fixators, the close proximity of the femur to the abdominal wall means that unilateral pin splintage (type I) is the most applicable frame type, although use of a modified type I frame using a full fixation pin in the distal femur and a curved connecting bar has also been described (Klause et al, 1990). A type I external skeletal fixator may resist axial, rotational and torsional forces well, but it has less resistance to bending forces. While major complications, such as implant failure, secondary fracture and non-union following femoral external skeletal fixator use are less common than the pin tract complications documented above, they can result in poor outcomes when they occur (Peirone et al, 2002; Kirkby et al, 2008).
While it is important to consider their limitations, some studies have demonstrated satisfactory fracture healing, with low complication rates and morbidity, when using external skeletal fixators for stabilisation of feline femoral fractures. Therefore, there may be a place for the use of appropriately applied unilateral external skeletal fixators in cats (Langley-Hobbs et al, 1996). However, it should be considered that in this study 71% of cases were stabilised with an intramedullary pin to complement the external skeletal fixator, so these favourable results may not be replicated with use of an external skeletal fixator alone.
While a Type I external skeletal fixator adequately counteracts rotation, shear and compression (Aron and Dewey, 1992), it has poor stabilisation against bending forces (Chao and Pope, 1982). Although, if the external skeletal fixator is used in combination with an intramedullary pin, all fracture forces can be adequately stabilised. In this configuration, a type I external skeletal fixator is applied to the lateral surface of the femur and an intramedullary pin is placed and allowed to protrude from the skin proximally. The pin is then bent laterally at the proximal extent and the connecting bar is attached to both the fixator pins and the intramedullary pin using clamps. A ‘tied-in’ configuration has been shown to prevent pin loosening, increase bending strength and increase the strength of the bone assembly compared with a simple construct without an intramedullary pin (Aron et al, 1991). A ‘tied-in’ pin is unable to migrate, decreasing the possibility of implant-associated neurapraxia (Withrow and Amis, 1977).
In external skeletal fixator configurations without an intramedullary pin, a minimum of two pins must be placed per fragment, causing muscle irritation, pain and limiting limb use (Whitehair and Vasseur, 1992). However, the use of an intramedullary pin in conjunction with the external skeletal fixator is advantageous in that, with the external skeletal fixator only being used to resist rotation and axial shortening, a two-pin fixator is usually sufficient, which limits complications associated with pin tract discharge as these can be placed in areas with less soft tissue coverage. The most proximal pin should be placed through the bone in the proximity of the greater trochanter and angled toward the lesser trochanter to engage the medial cortex, taking care not to penetrate the trochanteric fossa as this will result in reduced bone purchase (Langley-Hobbs et al, 1996). The most distal pin should be placed through the femoral condyles where there is minimal muscle mass and substantial bone stock (Langley-Hobbs et al, 1996).
Bone plates
Bone plates are applicable to almost all diaphyseal femoral fractures and are commonly used. They are typically applied on the lateral aspect of the bone, as the tension surface, and may be used in compression, neutralisation or as a bridging device. Bone plate fixation has been shown to result in superior clinical function, compared to the use of intramedullary pins or external skeletal fixators (Braden and Brinker, 1973), and is associated with lower complication rates in feline fracture management than the use of external skeletal fixators (Konning et al, 2013).
Bone plates may be placed via an open approach, typically the lateral approach to the femur, (Piermattei and Johnson, 2004) or via minimally invasive approaches. Although, when minimally invasive approaches are used, direct visualisation of the fracture fragments is not permitted and care must be taken to achieve appropriate alignment.
When used in isolation bone plates may be susceptible to cyclic failure caused by repeated bending, which is one of the major causes of implant failure (Johnson et al, 1998; Stiffler, 2004). One potential solution to this problem is to augment the construct with an intramedullary pin (Figure 6). The combination of a bone plate with an intramedullary pin is known as a plate-rod construct. The intramedullary pin is resistant to bending loads, irrespective of the direction from which they are applied, but has poor resistance to axial and rotational loads (Johnson et al, 1998). In a plate-rod construct, the intramedullary pin resistance to bending loads and the axial and torsional support of the bone plate are combined. In an in-vitro experiment, the addition of the intramedullary pin to the bone plate decreased strain on the plate twofold and subsequently increased the fatigue life of the plate-rod construct 10-fold compared with that of the plate alone (Hulse et al, 1997; Reems et al, 2003; Beale, 2004; Stiffler, 2004). The larger the diameter of the intramedullary pin, the greater the effect on plate strain with plate strain reducing by approximately 19%, 22% and 61% with the addition of an intramedullary pin occupying 30%, 40% and 50% of the medullary cavity respectively (Hulse et al, 2000).
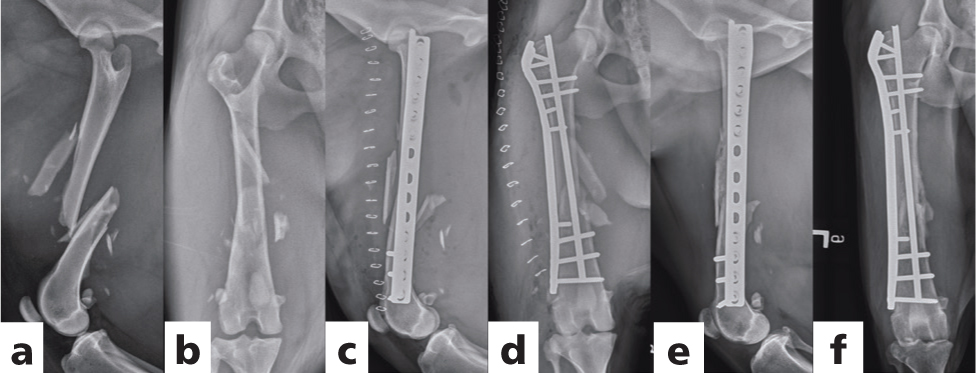
Plate-rod constructs may be used to stabilise a variety of fractures, ranging in severity from simple transverse to highly comminuted, (Reems et al, 2003) and very satisfactory results have been reported in both dogs and cats (Reems et al, 2003; Vedrine and Gerard, 2018). The technique can be applied via minimally invasive approaches with indirect fracture reduction, as well as open reduction with anatomic reconstruction. For a plate-rod construct, the intramedullary pin is placed first. As the pin engages the distal fragment it can be used to assist in distraction and reduction of the fracture. The intramedullary pin provides axial alignment and also maintains stability during plate application. However, the surgeon must take care to attain rotational alignment before placing screws in the bone plate. Only four-to-six cortices of purchase are needed in the proximal and distal fragments. Where bone stock is limited, either proximally or distally, the use of veterinary cuttable plates has been advocated when using a plate-rod construct, as the high number of screw holes per unit length may allow bicortical screw placement without interfering with the intramedullary pin (Vedrine and Gerard, 2018). When placing screws, assessing where the pin is likely to lie within the medullary canal and directing screws cranial or caudal to it is recommended, such that bicortical purchase can be obtained where possible. Where it is not possible to avoid the intramedullary pin, which is increasingly likely in narrower portions of the bone when a large pin has been used, monocortical screws are placed (Beale, 2004). This may be less of a problem in cats where the medullary to cortical ratio is higher. In this species, even when a large diameter intramedullary pin is placed, it may be easier to achieve bicortical purchase, particularly in the metaphyseal regions (Vedrine and Gerard, 2018).
When treating femoral fractures in young animals one must carefully consider the properties of the growing bone, such as the presence of physes, a thin and elastic cortex, and a thick periosteum that tends to separate from the cortex following trauma (Latte and Drape, 1991). In order to avoid fracture disease occurring (Bardet, 1987), it is essential to obtain stable fracture fixation which permits early use of the limb and maintenance of joint motion. While the use of the external skeletal fixator ‘tie-in’ approach has been advocated for use in skeletally immature patients (Peirone et al, 2002), the thin cortices of immature bone may predispose to transfixation pin pull-out. Careful placement of the intramedullary pin is necessary as placing it too close to the femoral neck may cause alterations in femoral head and neck development, as has been described both in humans (Gonzalez-Herranz et al, 1995) and dogs (Black and Withrow, 1979). Additionally, the development of painful, fistulous tracts at the level of the distal pin is common and causes a reduction in weightbearing (Peirone et al, 2002).
Because of the aforementioned complications, the use of bone plates for stabilising femoral fractures in juvenile patients is common. However, it has been proposed that the plating techniques commonly used to treat femoral fractures are poorly adapted to immature bone and carry a high risk of screw loosening due to the thin femoral cortices. Elastic plate osteosynthesis, where stress is distributed along the entire plate and pull out stress on screws is limited has been postulated to be more successful (Cabassu, 2001). Elastic plate osteosynthesis, using indirect reduction and veterinary cuttable plates placed as bridging osteosynthesis, has been proposed as an alternative with rapid callus formation and excellent functional results demonstrated (Cabassu, 2001). This avoids punctate fatigue and plate breakage, and as the screws are situated as far as possible from the fracture site (Figure 7), pull out forces are limited with loosening of screws not being identified as a complication in a series of 24 cases (Cabassu, 2001).
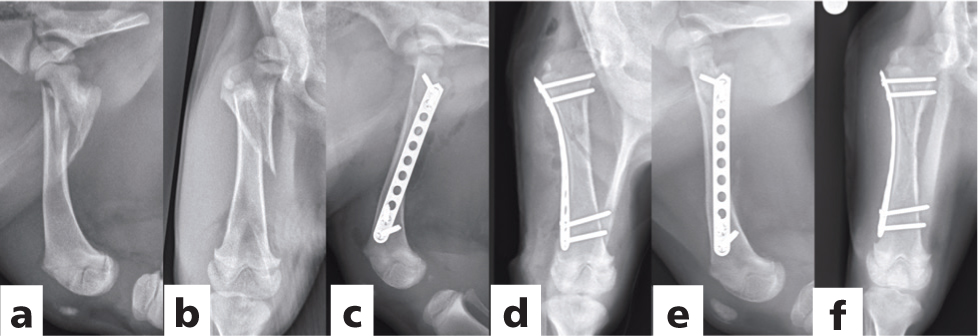
Interlocking nails
Because of their inherent mechanical and biological advantages, using interlocking nails to stabilise comminuted femoral fractures has become an attractive alternative to bone plating or plate-rod constructs (Duhautois, 2003; Horstman et al, 2004; von Pfeil et al, 2005; Lansdowne et al, 2007) (Figure 8). Compared with matching bone plates, interlocking nails have a relatively larger and more uniform area moment of inertia which allows them to effectively resist bending (Muir et al, 1995; Dejardin et al, 2006). Furthermore the intramedullary position of an interlocking nail, near the neutral axis of the bone, protects it from deleterious bending moments. In addition to resisting bending forces, interlocking nails provide axial and rotational stability via their locking bolts or bone screws.
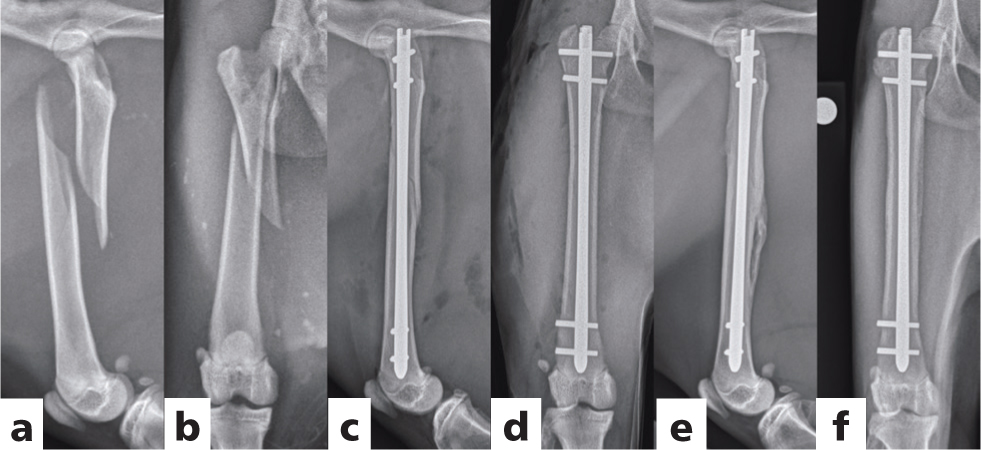
The interlocking nail is often used in people because it can be placed in a closed fashion with minimal disruption of soft tissue which protects the extraosseous blood supply, enhancing biological fracture fixation (Klemm and Borner, 1986). Similarly, in dogs, interlocking nails can be placed using minimally invasive techniques, a technique known as minimally invasive interlocking nailing (Dejardin et al, 2020).
Traditional interlocking nail designs were associated with moderate complications including prolonged healing times, excessive callus formation and a need for supplementary implants to overcome perioperative instability (Duhautois, 2003; Basinger and Suber, 2004; Goett et al, 2007; Suber and Basinger, 2008) as a result of mechanical deficiencies in their design, which resulted in mild persistent rotational and bending instability (Kaspar et al, 2005; von Pfeil et al, 2005; Dejardin et al, 2006; Lansdowne et al, 2007). This instability was attributed to the absence of a rigid mechanical interlock between the nail and the locking device (von Pfeil et al, 2005), resulting in acute instability in rotation and bending, commonly known as ‘slack’. To circumvent such limitations, angle-stable interlocking nails have been developed which eliminate both torsional and bending slack and provide superior clinical results (Lansdowne et al, 2007; Cabassu et al, 2009; Dejardin et al, 2009; Ting et al, 2009; Fauron et al, 2018; Marturello et al, 2019). There are two angle-stable interlocking nails currently available for use; the I-Loc, commercialised by BioMedtrix, Whippany, NJ and the Targon, commercialised by B Braun Vet Care, Germany. The I-Loc system is available in diameters from 3.0–8.0 mm, while the Targon system was designed for smaller patients and is only available in 2.5 mm and 3.0 mm diameters. The two systems also feature fundamentally different locking mechanisms.
The success rate of interlocking nailing using standard (not angle-stable) nails varies from 83–96%, with healing times ranging from 13 to 17 weeks (Durall and Diaz, 1996; Dueland et al, 1999; Duhautois, 2003; Diaz-Bertrana et al, 2005). Complications have been reported in up to 17% of cases treated with standard nails (Dueland et al, 1999). Although most are related to the nail being used when poorly indicated (for example, in the treatment of metaphyseal fractures with insufficient bone for screw insertion), some may be attributed to the limitations of the nail design itself including implant fracture and the development of delayed or non-union (Dejardin et al, 2020).
The clinical outcomes following the use of the I-Loc angle-stable interlocking nails have recently been reported in two prospective case series involving 100 and 25 consecutive traumatic fractures of the femur, tibia and humerus in dogs (Fauron et al, 2018) and cats (Marturello et al, 2019) respectively. In the canine study (Fauron et al, 2018), femoral fractures accounted for 64% of the cases and no complications were reported in femoral fractures specifically. Complications requiring additional surgery occurred in three cases involving tibial fractures including one infection necessitating implant removal, one fatigue failure of an undersized nail and one case of biological slack which occurred secondary to the bolts tearing through the medial cortex. In the canine study, minimally invasive techniques were used in 61% of fractures overall. The feline study (Marturello et al, 2019) included 12 femoral fractures. In contrast to in the canine study, only one third of cases in this study were performed using minimally invasive techniques. In terms of outcome, all cases were weight-bearing within 2 days and achieved full functional recovery with clinical union being achieved at a mean of 7.4 weeks. There were no major complications and only two minor complications.
Current studies evaluating the Targon nail (Bruckner, 2016) demonstrate slower healing with a clinical union rate of 61% at nine weeks. Additionally, the major complication rate using the Targon system has been reported to be higher at 18%. An important consideration for the Targon system, in addition to the limited inventory available, is that the bolts are designed such that they can only be placed in the diaphysis. This renders this a less versatile system as it cannot be used for epi- and metaphyseal fractures. However, for diaphyseal fractures it does remain an option.
Anatomically, cats are well suited for interlocking nail placement as they have relatively straight femurs which means that the nail can be well seated into the distal metaphysis without having to over-reduce the distal fragment (Larin et al, 2001). In contrast, in dogs, because of the caudal curvature of the distal femur, slight overreduction of the distal fracture fragment in the sagittal plane may be necessary, both when using interlocking nails and intramedullary pins, in order to allow deep seating of the implant and avoid penetration of the cranial cortex of the femur.
Supracondylar fractures
Supracondylar fractures are uncommon in dogs, accounting for 30% of distal femoral fractures and representing only 6% of all femoral fractures (Braden et al, 1995). Chondrodystrophic dogs do appear to be predisposed to these fractures because of their unique femoral anatomy (Lewis et al, 1993; Lidbetter and Glyde, 2000), so supracondylar fractures may be encountered more frequently in such breeds.
Supracondylar fractures involve the distal metaphyseal region of the femur, occurring at the transitional zone between the cortical bone of the diaphysis and the cancellous bone of the epiphysis (Lidbetter and Glude, 2000; O'Brien et al, 2006). While most supracondylar fractures remain isolated to the metaphysis, they can also propagate to involve the diaphysis or epiphysis and may be highly comminuted (Lewis et al, 1993; Beale, 2004). Most commonly, caudal displacement of the distal fracture segment is a result of the shape of the condyles and the muscular pull from the gastrocnemius muscle (Schrader, 1994; Simpson and Lewis, 2002; O'Brien et al, 2006; Piermattei et al, 2006).
Surgical treatment is recommended for almost all supracondylar femoral fractures in dogs and cats. Fractures may be anatomically reduced and stabilised, or indirectly reduced and treated using minimally invasive techniques. Regardless of the approach chosen, minimal dissection should be performed to decrease the chance of damaging the regional blood supply to the fragments, which is important for early bone healing (Beale, 2004). Owing to the paucity of bone stock distally, particularly with comminuted or associated epiphyseal fractures, supracondylar fracture reduction and stabilisation can represent a substantial challenge. To address this, numerous methods of fixation have been described including bone plates (Hickman, 1964; Crovace et al, 1992), interlocking nails (Fauron et al, 2018), crossed K-wires (Sumner-Smith and Dingwall, 1973), rush pins (Carney, 1952; Lawson, 1959; Campbell, 1976; Shires and Hulse, 1980), lag screws (Knight, 1956; Denny, 1993), intramedullary rods (Armistead and Lumb, 1952; Milton et al, 1980; Leighton, 1994; Stigen, 1999), external skeletal fixators (Piermattei and Flo, 1997), use of a single rush pin as an intramedullary nail (Robinson, 2000), and the use of polylactic acid biodegradable rods (Raiha et al, 1993). The use of crossed K-wires and rush pins is common in smaller animals, but is only appropriate for simple fractures as these techniques offer insufficient stability in comminuted fractures.
Bone plates applied to the distal femur are often the preferred method of fixation for supracondylar fractures, provided the distal fragment is of sufficient size to accommodate a minimum of two, preferably three, screws (Lewis et al, 1993; McLaughlin, 1993; Lidbetter and Glyde, 2000; Johnson et al, 2005). The ease of using traditional plates in this area is limited by their low screw-hole density and inability to be contoured in three-dimensions. As such plates specifically designed for the distal femur, featuring a hockey-stick profile, have been designed (Figure 9). Alternatively, the use of reconstruction plates, which may be contoured in three dimensions, can be considered although these are substantially weaker than conventional plates (Lewis et al, 1993; Piermattei et al, 2006). The use of conventional plates combined with over-reduction of the fracture may be also employed. Locking plates can be considered for application in this region, some of which are capable of three-dimensional contouring without losing their strength and stiffness. Examples include the string of pearls plate (SOP-Orthomed Ltd., Huddersfield, UK), the advanced locking plate system (ALPS – Kyon Veterinary Surgical Products, Boston, MA) and the FIXIN plate (intrauma, Rivoli, Italy).

Angle-stable interlocking nails are an attractive alternative to the use of bone plates, if sufficient bone stock is present within the distal fragment for the engagement of at least one of the two locking bolts (Reems et al, 2006). Modification of the distal tip of the interlocking nail, to shorten it, may be required to allow deep seating of the nail and optimal anchorage of both bolts in the cancellous bone of the distal metaphysis.
Distal femoral physeal fractures
Distal physeal fractures occur in skeletally immature patients, commonly between 4 and 11 months of age (Piermattei et al, 2006). These account for 60% of all distal femoral fractures and are the most commonly encountered physeal fracture in dogs (Kleine, 1971; Grauer et al, 1981; Marretta and Schrader, 1983; Braden et al, 2005), with a type II Salter–Harris fracture being the most commonly encountered in this species. In cats, type I Salter–Harris fractures are most commonly seen and early castration, resulting in delayed closure of the physis, has been postulated to increase the risk of this fracture in young adult cats (Smith, 1969; Houlton and McGlennon, 1992; Perry et al, 2013). Type III and IV fractures are rare in either species (Alcantara and Stead, 1975; Grauer et al, 1981; Marretta and Schrader, 1983; Parker and Bloomberg, 1984; Newton, 1989; Milton, 1993). Their treatment will be addressed in the section covering condylar fractures. Regardless of the fracture type, early reduction and stabilisation are important in preventing further physeal trauma and preserving remaining growth potential.
Although these fractures are rarely open, there is usually severe trauma to the surrounding tissues. The femoral diaphysis is usually markedly displaced through the tissues of the joint capsule and quadriceps muscle to lie in a subcutaneous position. Similarly to supracondylar fractures in adult patients, the pull of the caudal thigh muscles results in a caudoproximal displacement of the distal femoral fragment in relation to the diaphysis of the femur (Campbell, 1976). While fracture reduction may be difficult, particularly in more chronic cases (Schrader, 1994; Olmstead, 1995; Robinson, 2000; Piermattei et al, 2006), anatomic reduction will provide some rotational stability from the four pegs extending from the distal metaphysis, which align with four corresponding recessed regions in the distal epiphysis. Under-reduction, varus or valgus malalignment are to be avoided as they are associated with greater risks of implant migration, implant failure, patellar impingement, patellar luxation and malunion (Hardie and Chambers, 1984; Schrader, 1994; Piermattei et al, 2006).
Several methods of treatment have been described, including crossed K-wires, two rush pins and the use of polylactic acid biodegradable rods. Regardless of the stabilisation method chosen, attempts are made to avoid any configuration that would place compression across the physis. Fixation techniques that prevent physeal growth such as bone plates, lag screws and external skeletal fixators should be avoided. By far the most commonly used method of fixation employs at least two K-wires or small Steinmann pins applied in normograde fashion (Stigen, 1999; Simpson and Lewis, 2002; Beale, 2004) with the pins inserted from distal to proximal. The cross-over point of the pins must be located above the fracture line in order to provide sufficient stability. In order to achieve this, insertion of the pins at a 30–45° angle, relative to the anatomical axis of the femur, is recommended (Schrader, 1994; Sukhiani and Holmberg, 1997; Piermattei et al, 2006). Rush pinning has also been described with the pins being inserted at a steeper angle of 15–20°, with respect to the anatomical axis of the femur (Schrader, 1994; Harasen, 2001; Simpson and Lewis, 2002; Beale, 2004). This method is considered equally effective at neutralising the forces across the physis when compared to cross-pinning (Sukhiani and Holmberg, 1997). Following stabilisation of distal femoral physeal fractures, owners should be advised to monitor for limb shortening or limb deformity, which are rare but potential sequalae following physeal fracture.
Condylar fractures
Fractures involving the distal femoral epiphysis are infrequent in both young and adult animals, accounting for only 10–15% of all distal femoral fractures (Bardet and Vannini, 1998; Grauer et al, 1981; Unger et al, 1990; McLaughlin, 1993; Braden et al, 1995; Stead, 1998; Piermattei et al, 2006; Simpson and Lewis, 2002). In skeletally immature animals, fractures involving the distal femoral physis are described using the Salter–Harris classification system (Salter and Harris, 1963) with types III and IV representing those with an intra-articular component. Type III fractures involve the physis and extend through the epiphysis into the stifle joint. Type IV fractures extend from the metaphysis, through the physis and epiphysis, into the stifle joint. Condylar fractures are classified as unicondylar, or bicondylar depending on whether one or both femoral condyles are involved.
Fractures involving a single condyle are termed unicondylar. The medial condyle is more commonly fractured than the lateral, becoming separated from both the femoral shaft and the lateral condyle. Usually there is minimal displacement and the collateral ligaments remain intact and attached to the fractured condyle (Milton and Newman, 1983; Conte and Addison, 2019). Bicondylar fractures occur when the medial and lateral condyles fracture from each other, as well as being fractured at each of their junctions with the femoral shaft (Siliski et al, 1989). Bicondylar fractures are often described as ‘Y’ or ‘T’ fractures depending on the obliquity of the supracondylar component. Concurrent damage to the cruciate ligaments or menisci is not uncommon with bicondylar fractures and must be evaluated (McLaughlin, 1993).
Functional recovery after repair of intra-articular fractures of the distal femur depends on anatomic reduction, rigid internal fixation and early post-operative physical rehabilitation (Pond, 1975; Olmstead, 1984; Mize, 1989). Owing to the requirement for anatomic reduction, open reduction is often recommended for condylar fractures, and is certainly recommended for chronic fractures, comminuted fractures, or those where concurrent ligamentous injury is suspected. However, simple, minimally displaced fractures that are operated promptly can be reduced and stabilised through a closed approach. It should be noted that this requires intraoperative fluoroscopic or arthroscopic guidance to ensure that appropriate fracture reduction is attained (Guiot et al, 2012).
Internal fixation is recommended for repairing condylar fractures (Berzon, 1980; Milton and Newman, 1983). A parapatellar approach to the stifle joint usually provides adequate exposure for reduction and stabilisation. For fractures involving the lateral condyle, the parapatellar incision can be extended into a lateral approach to the femur to improve exposure of the supracondylar region. The arthrotomy also allows visualisation of the intra-articular fracture to ensure anatomic reduction of the articular surface. K-wires, pointed reduction forceps or vulsellum forceps can be used to maintain reduction while the intercondylar portion of the fracture is stabilised. Interframentary compression between the medial and lateral condyles is important to produce rigid stability and allow early postoperative use of the limb (Francis and Johnson, 1990) which is best achieved by placing a transcondylar lag screw perpendicular to the fracture line. Care must be taken to ensure that this screw does not enter the condylar fossa and cause damage to the cruciate ligaments. Although two screws provide better rotational stability, there is often insufficient room for placement of two screws in this area, and rotation around the lag screws will be prevented when the supracondylar portion of the fracture is stabilised. Once the condyles are anatomically reduced and the lag screw is placed, the supracondylar portion of the femur is repaired. In skeletally immature animals, compression across the physis is avoided to limit the risk of premature closure and subsequent limb shortening or angular deformity and crossed pins, intramedullary pins or a modified rush pin technique are commonly used (McLaughlin, 1993). In skeletally mature animals, a variety of stabilisation techniques can be considered, depending on fracture configuration, including crossed pins, supracondylar lag screws and rush pins, and bone plates.
Bicondylar fractures, or intercondylar fractures, are complex and frequently associated with severe soft tissue trauma, particularly affecting the cruciate ligaments and menisci (Beck et al, 1992; Johnson et al, 2005; O'Brien et al, 2006; Piermattei et al, 2006). Advanced imaging with CT is strongly recommended for such fractures to fully characterise the fracture configuration and assist with preoperative planning.
The vast majority of bicondylar fractures require an open approach due to the commonly associated comminution and soft tissue injuries requiring assessment. A parapatellar approach to the stifle joint is usually adequate. While osteotomy of the tibial tuberosity and elevation of the quadriceps has been used to increase exposure (Mize et al, 1982), this approach is associated with significant morbidity and is only used when sufficient exposure cannot be achieved any other way. The condyles are reduced and held in position with K-wires or bone-holding forceps. Compression between the condyles is created by placing one or more intercondylar lag screws.
Fractures where comminution exists in the articular surface may be difficult to repair. Osteochondral fragments are reduced and stabilised if possible, in order to minimise development of osteoarthritis. K-wires are often used to stabilise small fragments but do not generate interfragmentary compression. Lag screws can be used to stabilise larger fragments (Milton and Newman, 1983; Kaderly, 1989). The head of the screw is counter-sunk below the surface of the articular cartilage to minimise cartilage damage during joint motion (Kaderly, 1989). Alternatively, headless screws can be used including the Herbert bone screw (Zimmer Inc., Warsaw, IN) and the Acutrak screw (Acumed, Hillsboro, OR). The use of bioabsorbable implants to reattach osteochondral fragments has also been reported (Claes et al, 1985; MaKela et al, 1989). Fragments that cannot be stabilised are discarded (Milton and Newman, 1983).
Once the joint surface has been reconstructed, the remaining supracondylar fracture is repaired. Crossed pins, crossed lag screws, Steinmann pins and rush pins can be used to stabilise fractures where no comminution exists. External fixators may be used to augment primary stabilisation if necessary (Toombs et al, 1989) and have also been used as the primary means of fracture stabilisation, particularly in open or comminuted supracondylar fractures. However, external fixators do not allow the creation of interfragmentary compression between condyles, so these are generally not recommended as a first choice for treatment. In larger animals, or those with comminuted fractures, a bone plate is preferred to repair the supracondylar fracture as this can achieve more rigid fixation and allow earlier return to limb function, which will subsequently maintain range of motion and improve joint function (Olmstead, 1984).
Trochlear femoral fractures
Typically, the aforementioned condylar fractures do not affect the femoral trochlea (Piermattei and Flo, 1997). However, there are some articular fractures of the distal femur that do not conform to the typical ‘T’, ‘Y’ or ‘L’ configuration. These fractures differ from the more commonly encountered fractures, in that the trochlear component is oblique, transverse or comminuted, and not perpendicular to the condyles. In these cases, one or several fracture lines may cross the femoral trochlea in an oblique or horizontal pattern and small or large trochlear fragments can be dislodged from the femur (Chico et al, 2001).
While similar principles of repair may be applied to those described for condylar fractures, these types of fracture pose a challenge. In order to restore adequate support for the stifle extensor mechanism, it is imperative to maintain the integrity of the trochlea. A suboptimal surgical repair may lead to delayed or non-union, functional impairment of limb use, and the development of stifle osteoarthritis. Indeed, the prognosis has been shown to be more guarded in articular fractures that involve the trochlea, although it has been postulated based on the results of two studies (Hardie and Chambers, 1984; Chico et al, 2001) that cats may have a better prognosis than dogs. Reasons put forward for this include, facilitated reduction of the fracture, ease of performing postoperative rehabilitation and their lighter body weight (Chico et al, 2001). Although, the difficulty in performing gait assessment in cats may also lend a false sense of security here.
Complications
The most common complications, specific to proximal femoral fractures, include:
- Premature physeal closure
- Resorption of the femoral head or neck
- Inadequate reduction and malunion
- Altered coxofemoral development with subsequent osteoarthritis (Daly, 1978).
Less common complications include (Daly, 1978; Newton, 1989):
- Implant failure
- Implant migration
- Non-union
- Sciatic neurapraxia
- Infection.
When considering femoral shaft fractures, the femoral diaphysis is the most common long bone affected by non-union and osteomyelitis, with other potential complications including malunion, sciatic neurapraxia and quadriceps contracture (Cechner et al, 1977; Caywood et al, 1978; Smith et al, 1978; Hunt et al, 1980; Fanton et al, 1983). Many of the complications reported following diaphyseal femoral fracture repair relate to the inappropriate use of intramedullary pins, so can be largely avoided. One study reported an incidence of 14.5% sciatic nerve entrapment secondary to treatment via intramedullary pinning (Fanton et al, 1983). In a review of femoral shaft fractures in young dogs treated with intramedullary pins (Black and Withrow, 1979), seven out of eight cases developed characteristic alterations of the coxofemoral joint postulated to be secondary to epiphyseal trauma, ischaemia and mechanical impairment of normal growth from intramedullary pin presence. Less common complications following femoral diaphyseal fracture repair include implant loosening, implant failure and infection, the incidence of which can be reduced by use of meticulous surgical technique and appropriate implant selection.
Complications secondary to supracondylar fracture repair include implant-associated lameness caused by implant interference with the patella, malunion, patellar luxation and quadriceps contracture (Lidbetter and Glyde, 2000). Complications associated with condylar fractures are often associated with inadequate reduction and/or fixation. These include delayed union or non-union, patellar luxation and quadriceps contracture (Francis and Johnson, 1990; Beale, 2004). The development of osteoarthritis is anticipated after surgical repair of articular distal femoral fractures, but is minimised by meticulous surgical technique, anatomic reduction, rigid fixation and early postoperative physical rehabilitation (McLaughlin, 1993).
Prognosis
Overall, the prognosis for complete functional recovery following most femoral fractures is good to excellent, providing that effective mechanical stability is achieved and an optimal healing environment is preserved (Guiot et al, 2012). Although the prognosis associated with condylar fractures is less favourable than a nonarticular femoral fracture, a good clinical outcome can still be achieved, especially if anatomic reconstruction, rigid fixation and physical rehabilitation are all implemented (McLaughlin, 1993; Chico et al, 2001).
For all femoral fractures, weight-bearing should be encouraged immediately after surgery and fractures should be stabilised adequately so that this is acceptable. Early return to function reduces the chance of fracture disease and encourages early callus formation. Time to clinical union may vary from 2–16 weeks, depending on the age of the patient, the type of fracture, method of repair, surgical approach used, compliance of the owner and compliance of the animal.
Conclusion
Femoral fractures are commonly encountered in dogs and cats and are generally not amenable to treatment with external coaptation. In preparation for surgery, careful preoperative assessment and planning is required if optimal results are to be obtained. Radiographic assessment is required as a minimum, while complex fractures will benefit from advanced imaging using computed tomography. Femoral fractures can be treated using the principles of biological osteosynthesis, with minimally invasive or look-but-do-not-touch approaches, or by anatomic reduction and fixation. Stabilisation methods chosen vary extensively, depending on the anatomic location of the fracture. However, regardless of fracture location and type, the prognosis for a comfortable, functional limb following treatment is generally good-to-excellent.
KEY POINTS
- Computed tomography is a valuable diagnostic tool for assessment of the proximal and distal femur, particularly in cases of comminution, articular involvement or fissures.
- Femoral fractures are generally not amenable to treatment with external coaptation due to the anatomy of the femur and the surrounding soft tissue envelope.
- The use of minimally invasive approaches, where appropriate, expedites healing and is associated with low patient morbidity.
- The use of intramedullary pins in isolation, or stack pinning, to stabilise fractures of the femoral diaphysis is associated with an unacceptably high complication rate and cannot be recommended.
- External skeletal fixator use for stabilisation of femoral diaphyseal fractures is associated with significant inherent mechanical and biological limitations.
- Animals presenting with femoral condylar fractures must be assessed for concurrent damage to the cruciate ligaments and menisci.