Most neurological spinal cord presentations in small animal practice are caused by non-traumatic diseases, such as intervertebral disc disease (Olby et al, 2003; Marioni-Henry et al, 2004; Fluehmann et al, 2006). However, fractures and luxations of the vertebral column can cause serious spinal cord injury (Olby et al, 2003; Bruce et al, 2008; Bali et al, 2009). In general, vertebral fractures and luxations represent approximately 10% of all neurological presentations in dogs and cats (Marioni-Henry et al, 2004; Fluehmann et al, 2006; Simpson et al, 2009).
Fractures or luxations of the vertebral column are a common consequence of external trauma (McKee, 1990; Olby et al, 2003; Marioni-Henry et al, 2004). More than half (60%) of spinal cord injuries, in dogs not affected by intervertebral disc disease, are a result of motor vehicle accidents (Simpson et al, 2009). Specifically, road traffic accidents are the cause of 41–63% of vertebral luxations and fractures (Bruce et al, 2008; Bali et al, 2009; Hettlich et al, 2010).
Other reported traumatic events that can cause vertebral fractures and luxations are falls from height (in cats and toy breed dogs), dog fights, gunshots and falling objects. Toy breeds can develop atlantoaxial instability secondary to minor trauma as a result of congenital malformations of the dens and associated ligaments (Dewey et al, 2013). Pathological vertebral fractures caused by neoplasia, infection or metabolic disease represent a minority of the cases (Rusbridge et al, 1999; Cabassu and Moissonnier, 2007; Jeffery, 2010). Iatrogenic causes, such as subluxation and fracture of the articular facets following lumbosacral laminectomy (Moens and Runyon, 2002; Kinzel et al, 2004), and idiopathic causes, such as stress fractures in working dogs (Jeffery, 2010) (Figure 1), are also rare.
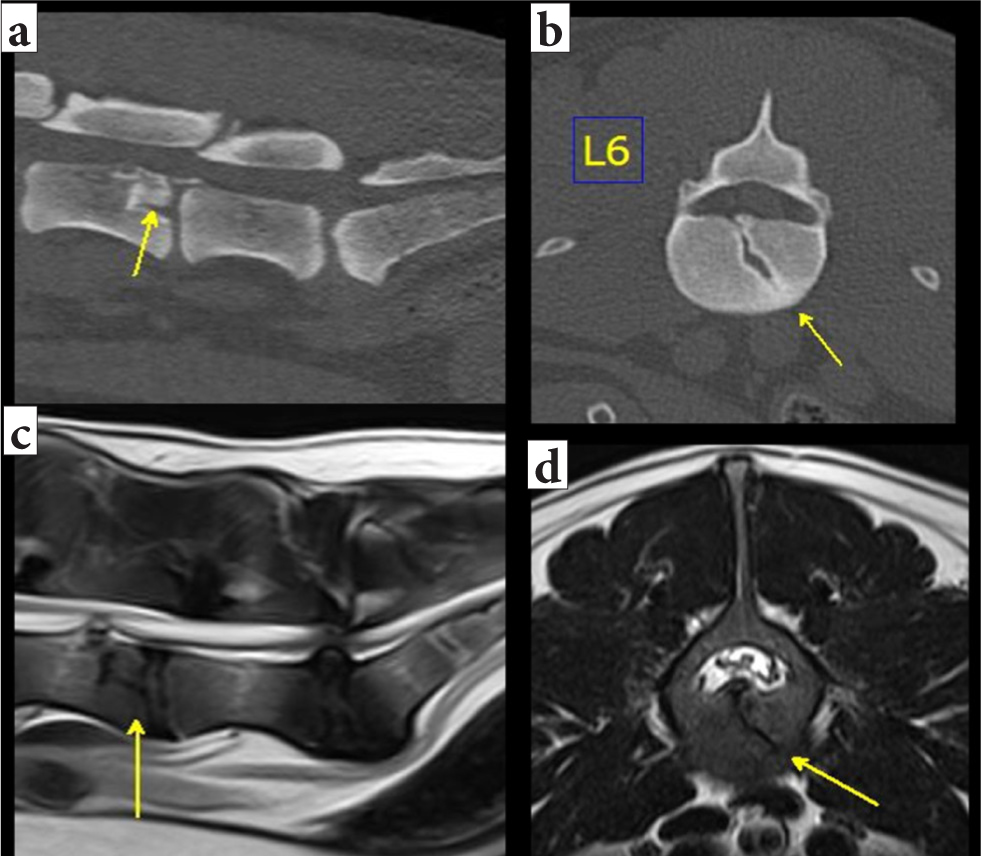
How to approach patients with a possible spinal fracture or luxation in three steps
- Step 1: Airways-breathing-circulation (ABC) assessment, stabilisation and immobilisation
- Step 2: Neurological examination
- Step 3: Analgesia, sedation or anxiolysis.
Step 1: Airways-Breathing-Circulation assessment, stabilisation and immobilisation
Performing rapid and accurate initial triage and stabilisation (Figure 2), followed by a neurological examination, is pivotal for successful management of patients with vertebral fractures and luxations (Jeffery, 2010).
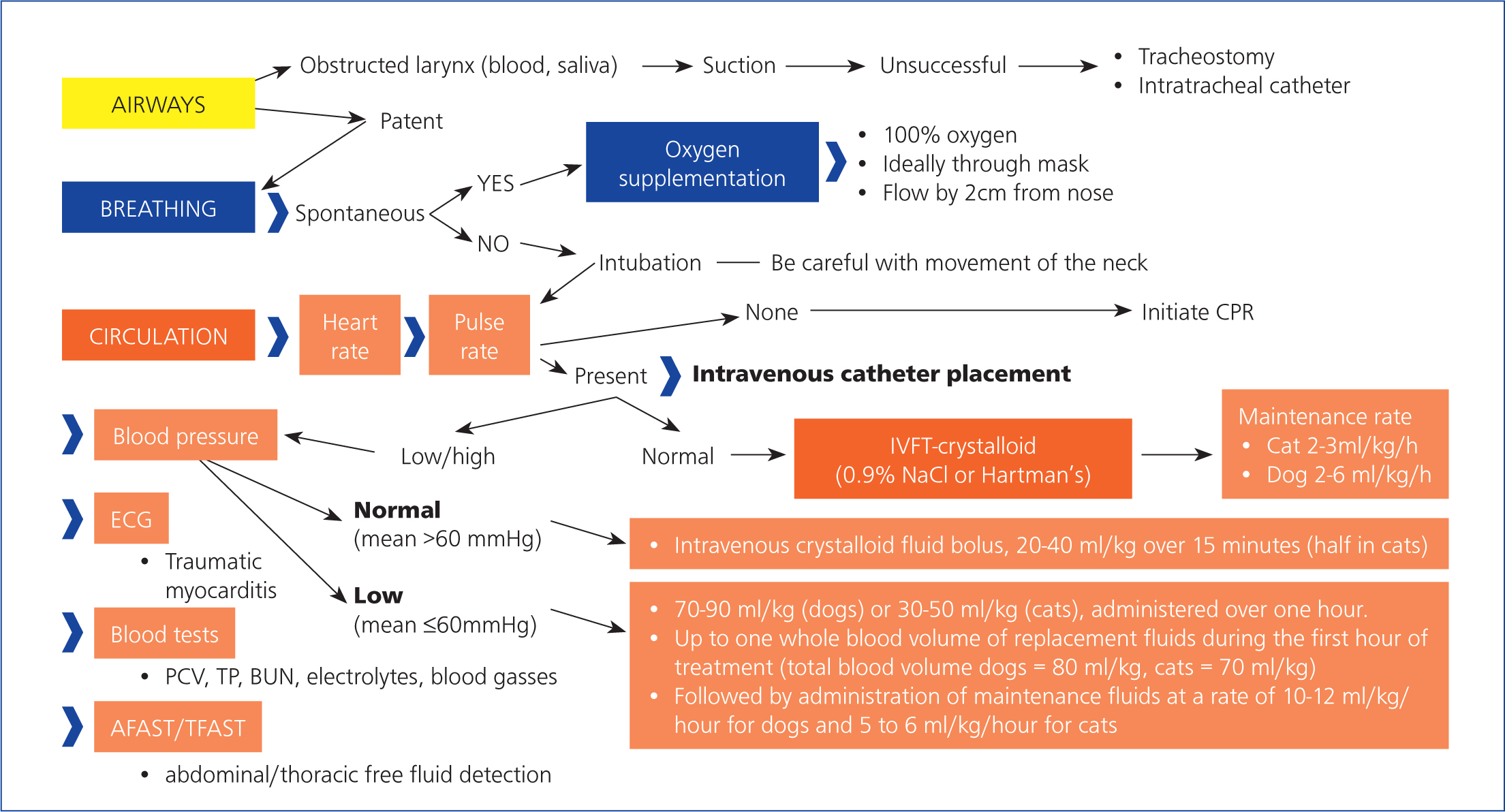
The initial systemic stabilisation should maintain adequate blood consequence of thoracic trauma is traumatic myocarditis, recognised flow and oxygenation. This is achieved by intravenous fluid therapy and oxygen supplementation, to prevent secondary spinal cord injury following the initial traumatic event (primary injury) (Olby, 2010).
Depending on the level of the vertebral column injury (cervical, thoracic, or lumbar vertebrae), secondary spinal cord injury can result from loss of regulation, of local or systemic blood pressure (Tator and Fehlings, 1991; Krassioukov and Claydon, 2006) and reduced spinal cord blood flow, caused by vascular damage. Local perfusion of the spinal cord is reduced by increased intraspinal cord pressure, caused by cytotoxic oedema and haemorrhage and by a failure of autoregulatory mechanisms. Decreased perfusion initiates and perpetuates secondary damage (Olby, 2010). Therefore, it is critical to maintain systemic blood pressure and oxygenation within normal thresholds. Both hypotension and hypoxaemia can greatly exacerbate the severity of an injury (Jeffery and Blakemore, 1999; Olby, 1999). There is a close relationship between the perfusion of the injured spinal cord and the outcome of the patients, which highlights the crucial importance of maintaining appropriate blood flow (Tator and Fehlings, 1991; Olby, 2010).
It is crucial to address potential systemic abnormalities as well. Thoracic trauma (such as pneumothorax, rib fractures, pulmonary contusion) occurs in 15–35% of patients with vertebral column injury (Turner, 1987; Bruce et al, 2008) (Figure 3). It is important to bear in mind that pulmonary contusions can worsen in the first 24–48 hours after the traumatic event, which may be a significant concern in terms of anaesthesia (Ganie et al, 2013). A less common by electrocardiogram (ECG) changes, including arrhythmias such as ventricular premature complexes, ventricular tachycardia, atrioventricular block, atrial tachycardias, and bundle–branch blocks. Retrospective veterinary studies evaluating presumed myocardial injury in dogs with thoracic trauma reported a range of 10–96% for traumatic myocarditis. A minority of these animals experienced arrhythmias. Usually, traumatic myocarditis is a self-limiting process (Murphy and Nakamura, 2019).

Abdominal trauma is reported in 6–15% of vertebral column injury patients (Turner, 1987; Bruce et al, 2008). This may result in haemoperitoneum, uroperitoneum, gastrointestinal or hepatobiliary injuries.
Concurrent fractures to the pelvis and appendicular skeleton are reported to be present in 14–48% of animals with vertebral fractures or luxations (Turner, 1987; Bruce et al, 2008). Concurrent injuries (such as pneumothorax, appendicular fractures or haemoperitoneum) are more common in cats than dogs (83% in cats, 66% in dogs) (Bali et al, 2009).
Injuries to the vertebral column can result in instability and unstable vertebral fractures are dynamic. In order to prevent spinal cord damage and worsening of the neurological status, gentle manipulation or immobilisation techniques such as bandaging (Figure 4) or splinting (Figure 5) should be applied promptly. Delayed immobilisation can result in devastating or fatal consequences, especially in case of cranial cervical damage, as seen in atlantoaxial injury (Figure 6) and atlantooccipital injury (Figure 7) (McKee, 1990; Hawthorne et al, 1999).
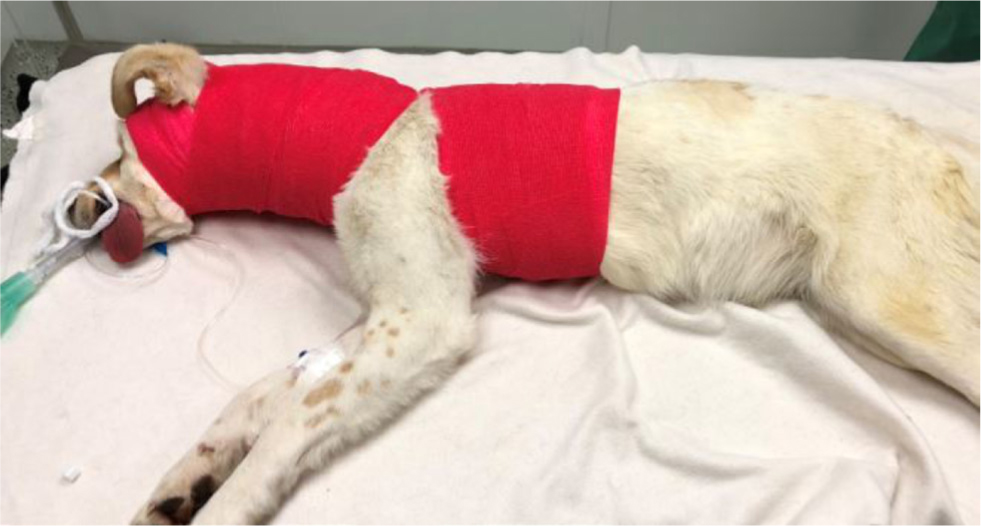
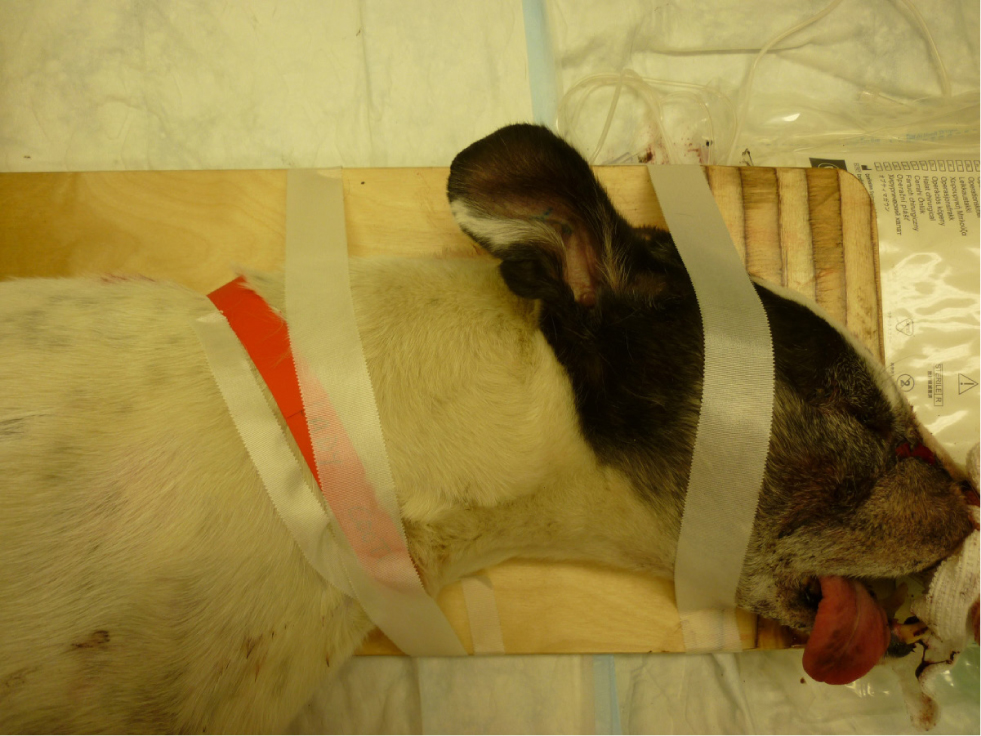

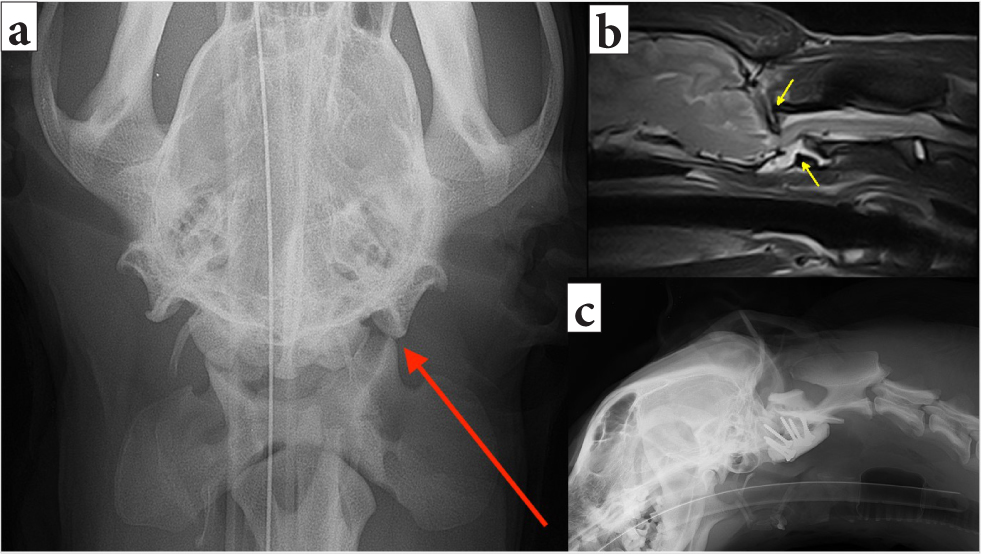
Step 2: neurological examination
Animals with spinal trauma and vertebral fractures or luxations can present with a range of clinical signs, depending on the location and severity of the injury, and show clinical presentations such as spinal discomfort, paresis, paralysis. These can be similar to those in patients with other spinal cord injuries (such as intervertebral disc disease and fibrocartilaginous embolism) (Olby et al, 2003; Bruce et al, 2008; Jeffery, 2010), Figures 8 and 9 show imaging findings in animals presenting both with no neurological deficits and with spinal discomfort in the neck and lumbar area respectively.
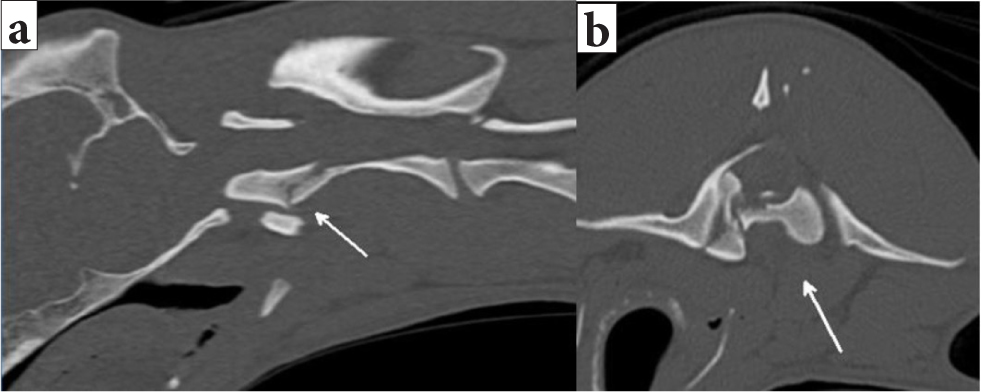
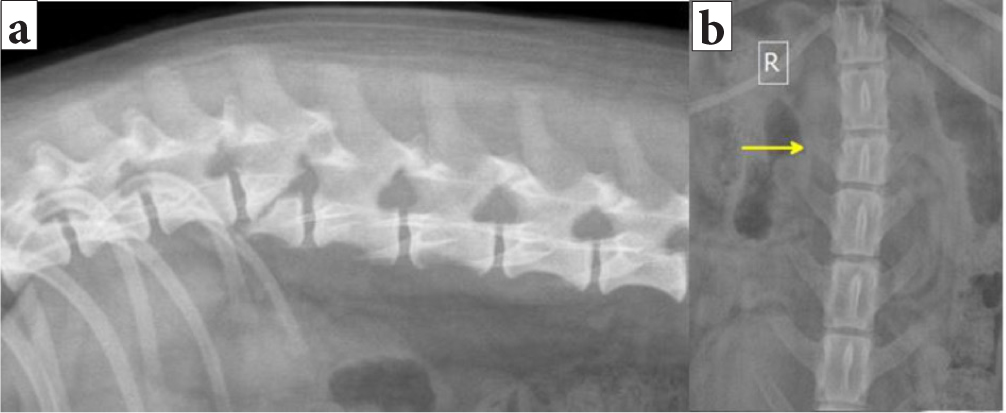
Rapid screening to assess an animal with a vertebral fracture or luxation is initially sufficient. To avoid potential iatrogenic damage to the spinal cord during the manipulation of the patient, which can happen during postural reaction testing, a full neurological examination might not be necessary (Figures 10 and 11) (Penderis, 2008; Jeffery, 2010).
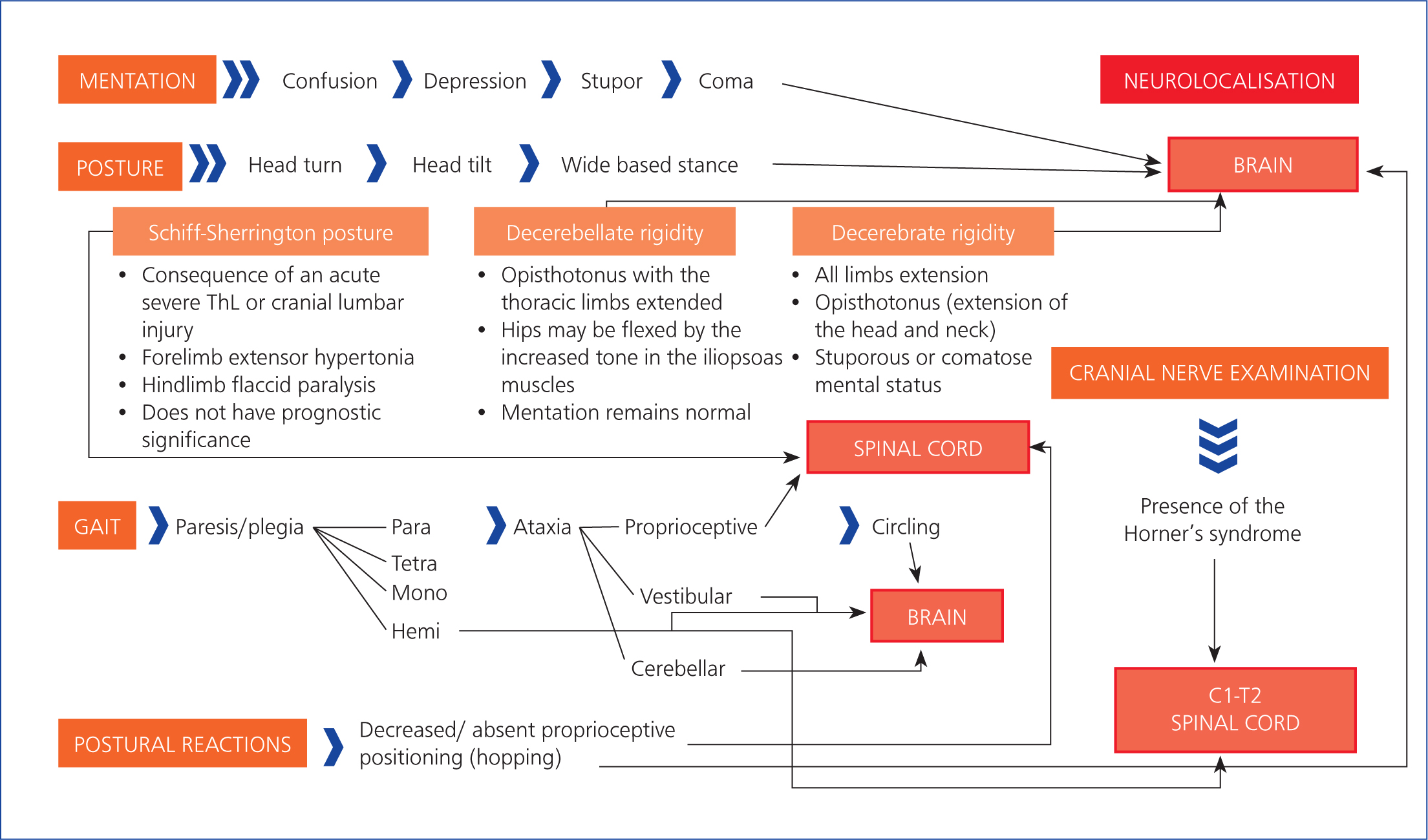
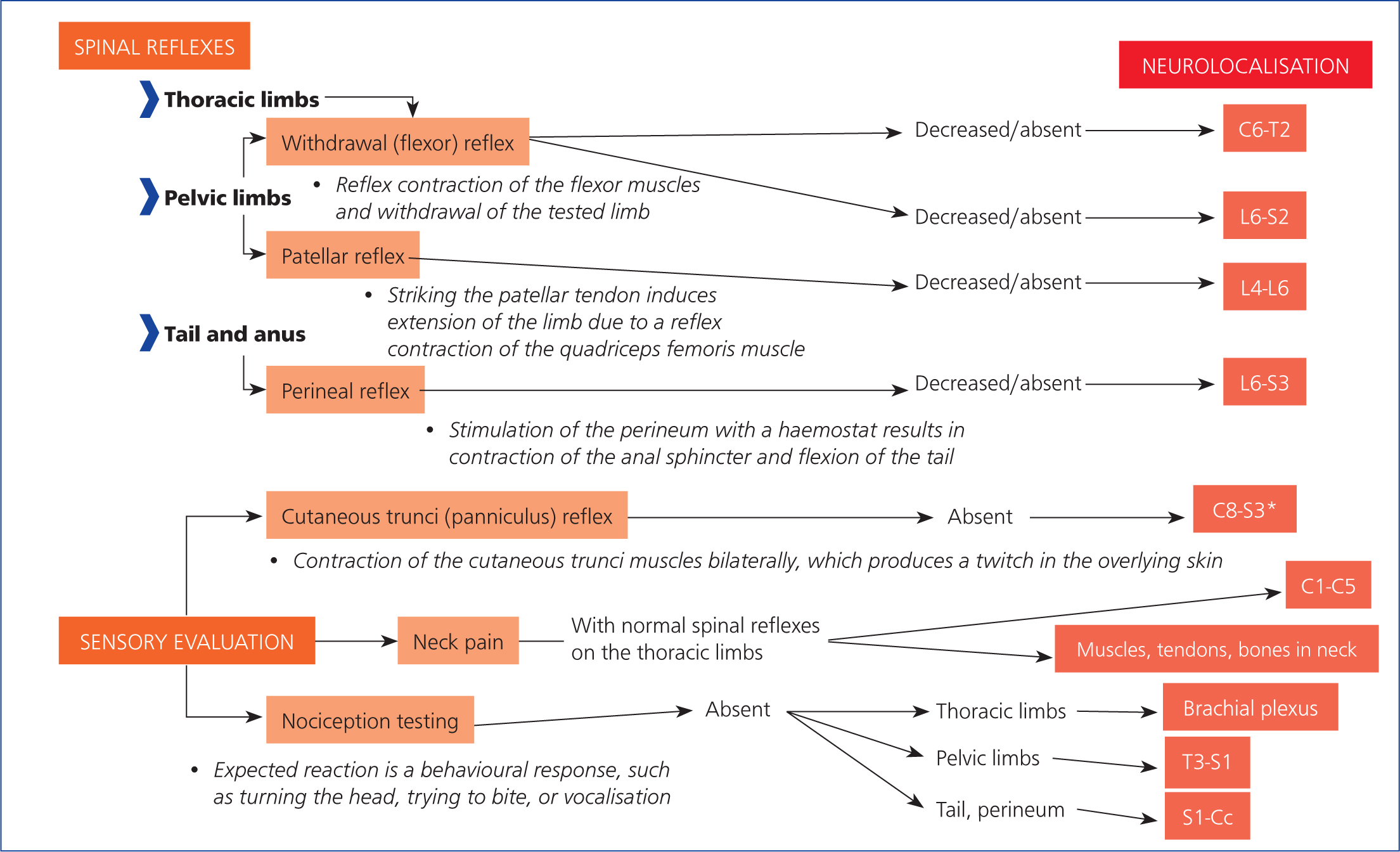
The neurological examination aims to localise the lesion(s) affecting the nervous system and determine their extent and severity (Penderis, 2008) (Figures 10 and 11). Initial grading, at the time of presentation, remains the most important clinical feature affecting the prognosis or outcome of a spinal cord injury (Grasmueck and Steffen, 2004; Penderis, 2008). The decrease or absence of muscle tone and segmental spinal reflexes, caudal to a severe thoracolumbar spinal cord injury, may indicate spinal shock. This predominantly occurs in cases of fibrocartilaginous embolism, intervertebral disc herniation or acute non-compressive nucleus pulposus extrusion, but can occur with other diseases (Full et al, 2016). In a patient with spinal shock, a loss or decrease in segmental spinal reflexes caudal to a lesion in the T3–L3 spinal cord segments occurs despite the reflex arcs remaining physically intact (Smith and Jeffery, 2005; Negrin et al, 2018). Spinal shock does occur in animals and is important to accurately localise lesions to manage these cases (Smith and Jeffery, 2005). It is generally considered to be short-lived in dogs and cats. Existing evidence suggests that pelvic limb withdrawal reflexes may be decreased as a result of spinal shock, following an upper motor neuron spinal cord lesion, for periods of 12–48 hours (Smith and Jeffery, 2005; Negrin et al, 2018).
Step 3: analgesia, sedation and anxiolysis
Animals that have incurred vertebral fractures or luxations are expected to be in pain (Jeffery, 2010). Preventing pain in these cases is crucial because pain initiates a series of neuroendocrine events, leading to hypothalamic–pituitary–adrenal gland axis activation, with subsequent release of proinflammatory cytokines and catecholamines. These processes result in altered immune function, as well as cardiorespiratory changes. Pain also decreases subcutaneous oxygen tension, impairing the wound healing (McGuire et al, 2006). A similar process results in altered spinal cord blood flow and oxygenation, potentiating secondary spinal cord damage (Olby, 2010).
Multiple types of medications can be used for pain relief, from opiates, to ketamine (0.2–0.5mg/kg IV), lidocaine (1–2mg/kg IV), and non-steroidal anti-inflammatory drugs (NSAIDs) such as meloxicam (0.2mg/kg IV in dogs, 0.1mg/kg IV in cats) or carprofen (4mg/kg IV in dogs for 24 hours, 4mg/kg IV in cats as a single dose) (Jeffery, 2010). Individual cases may require anxiolysis (with acepromazine or trazodone) or sedation.
Opiate administration is appropriate for use in the early post-injury period in most instances (Jeffery, 2010). Opioid agonists, such as fentanyl (0.002–0.005mg/kg IV in dogs, 0.005–0.01mg/kg IV in cats) or methadone (0.1–0.4mg/kg in dogs, 0.1–0.3mg/kg IV in cats), are ideal for use in critical patients because they are highly effective as pain relief and are safe (overdoses are reversible by naloxone) (Pascoe, 2000). Opioids provide a variable duration of action, depending on the specific drug, and can also be delivered as a continuous rate infusion (such as fentanyl). Cardiovascular side-effects of the opioids include vagally-mediated bradycardia, which is most pronounced with the more potent opioids (such as fentanyl) (Pascoe, 2000; Mathews et al, 2014). Loss of appetite following opiate administration can be a problem, but it is usually short lasting (Jeffery, 2010).
Ketamine, an N-methyl-D-aspartate (NMDA) receptor antagonist, possesses moderate analgesic qualities. In addition, NMDA receptor antagonists modulate pain at the level of the spinal cord, reducing central sensitisation and wind-up. Analgesic and sedative effects are best achieved through use in combination with an opioid. Ketamine has dose-dependent sympathomimetic activity, so caution should be exercised and the dose should be reduced in animals with cardiac disease or hypertension (Diaz et al, 1976; Suleiman et al, 2012; Quandt, 2013). Ketamine increases the cerebral metabolic rate and is not recommended in patients who are, or may be, at risk of elevated intracranial pressure, such as those with traumatic brain injury (Zeiler et al, 2014).
When pain is unresponsive to opiates and ketamine, the use of lidocaine can be justified. However, its effectiveness in cases of spinal pain has not been assessed. Lidocaine should be used in normovolaemic patients only, because of its depressant effect on cardiovascular function and subsequent contribution to hypotension. Lidocaine is not recommended in cats on account of its neuroexcitatory effects (Shaffran, 2013).
Agents such as NSAIDs are beneficial because of the anti-inflammatory response and analgesia provided by their cyclooxygenase-2 inhibiting activity. However, potential side effects arising from their cyclooxygenase-1 inhibition need to be carefully considered, for example, there can be reduced renal blood flow and interference with gastric mucosal integrity and platelet function (Mathews, 2001; Mathews et al, 2014). In addition, NSAIDs must be avoided in dehydrated or hypovolaemic animals, those with organ dysfunction or other comorbidities, as well as in animals receiving other medications that can potentiate the side effects, including diuretics, nephrotoxic drugs and corticosteroids (Mathews et al, 2014).
Acepromazine can be a useful anxiolytic agent for animals with spinal disease. The use of this agent should be limited to normovolaemic and normotensive animals (Quandt, 2013), as it has hypotensive effects.
Trazodone, an antidepressant and serotonergic agonist-antagonist, can also be considered for treatment of anxiety in patients able to take oral medication (Gruen et al, 2014).
Benzodiazepines (such as diazepam and midazolam), are potentially detrimental to animals with unstable spinal lesions on account of their effects on skeletal muscle relaxation, which subsequently reduces the splinting effects of the epaxial muscles (Quandt, 2013).
Alpha-2 adrenoceptor agonists (such as medetomidine or dexmedetomidine) should be used with extreme caution, as their vasoconstrictive effects may further compromise blood flow. Alpha-2 agonists also provide muscle relaxation so, as with benzodiazepines, these agents should be used with caution in animals with an unstable vertebral column (Quandt, 2013).
In summary, inhibiting the neuroendocrine stress response to avoid secondary spinal cord injury, maintaining tissue perfusion, allowing a restful sleep, improving appetite, and managing peripheral and central sensitisation are the main aims of pain management in animals with vertebral fractures and luxations. Increased care should be taken to immobilise the patient once pain relieving treatment has commenced (Jeffery, 2010).
How to evaluate the severity and prognosis after initial stabilisation and examination?
Veterinary literature discussing the exact outcome predictors for vertebral fractures and luxations is scarce. Nevertheless, information can be extrapolated from research on other spinal cord injuries, especially intervertebral disc extrusions.
Possible prognostic indicators extrapolated from the causes of other spinal cord injuries, such as intervertebral disc extrusions and fibrocartilaginous embolism, include:
- Traumatic injury itself (Olby et al, 2003; Penderis, 2008)
- Initial presence or absence of nociception (Olby et al, 2003; Penderis, 2008)
- Duration of the disease (Penderis, 2008)
- Localisation (Jeffery, 2010; Penderis, 2008)
- Lesion extent (Tartarelli et al, 2005; Penderis, 2008)
- Occurrence of progressive myelomalacia (Penderis, 2008)
- Age (Bali et al, 2009)
- Bodyweight (Olby et al, 2003; Bali et al, 2009)
- Historical or present concurrent diseases (Penderis, 2008).
Traumatic spinal cord injury, accompanied with loss of nociception, is a negative prognostic factor. In a study of dogs with spinal cord injuries, it was reported that none of the dogs with paraplegia and loss of nociception following spinal trauma regained nociception, compared to 58% of the dogs with intervertebral disc disease, which regained nociception and ability to walk (Olby et al, 2003; Penderis, 2008). Nociception is a critical prognostic factor (Olby et al, 2003; Penderis, 2008; Shaw et al, 2017). The absence of nociception in the limbs caudal to the spinal cord lesion indicates poor prognosis for return of function, regardless of the type of spinal cord injury, be it traumatic, degenerative or vascular (Jeffery, 2010; Olby et al, 2003; Penderis, 2008). In the study by Olby et al (2003) only 12% of dogs with traumatic thoracolumbar spinal cord injuries and lack of nociception regained ability to ambulate, despite a persistent lack of nociception. These dogs did not regain normal spinal cord function. However, they generated ‘spinal walking’, which can be described as coordinated reflex walking patterns that develop within the spinal cord, without any input to or information from upper motor neurons, after thoracolumbar spinal cord injury (Gallucci et al, 2017).
Reliable data supporting the successful treatment and outcome of animals without nociception, in dogs and cats with vertebral fractures and luxations, are scarce (Jeffery, 2010; Gallucci et al, 2017). In patients where treatment was initiated, a lack of improvement or complications secondary to paraplegia, such as urinary tract infection or progressive myelomalacia (Grasmueck and Steffen, 2004; Bruce et al, 2008; Bali et al, 2009), resulted in euthanasia.
In patients with spinal cord injuries, similarly to those caused by vertebral fractures or luxations (such as intervertebral disc extrusions or fibrocartilaginous embolism), prolonged loss of nociception (particularly when greater than 48 hours) is another feature indicating that treatment is less likely to be successful (Penderis, 2008). The importance of the duration of clinical signs is supported by experimental studies of spinal cord injuries, which revealed a direct correlation between the time of decompression after injury and clinical outcome (measured either as a return to ambulation or use of somatosensory evoked potentials) (Delamarter et al, 1995; Carlson et al, 2003). However, some clinical studies, including Macias et al (2002) and Olby et al (2003; 2004), showed that the outcome in dogs with less severe signs (ambulation, intact proprioception, nociception) on presentation was good, irrespective of the duration of the clinical signs, although, a longer duration did correlate with a longer recovery period. The speed of onset of the neurological clinical signs also correlated with a worse outcome (Macias et al, 2002; Olby et al, 2004). In another retrospective study of thoracolumbar disc extrusion and paraplegic dogs, there was no correlation between the duration of clinical signs and outcome, or rate of onset (Ito et al, 2005).
Vertebral fractures and luxations that affect the spinal cord intumescences are of particular significance because these regions of the spinal cord contain the grey matter and nerves that supply the limbs, urinary bladder and anus. Injury to these regions (vertebrae C4–T3 and L3–L5) carries a greater risk of permanent paralysis of the innervated regions, because of a greater susceptibility of the grey matter to injury (Penderis, 2008; Jeffery, 2010). The ability of plastic responses to ameliorate the functional deficits that follow spinal cord injury is more restricted for grey matter lesions than white matter lesions (Jeffery and Blakemore, 1999). It is reported that severe lesions of L5 vertebra were especially associated with poor prognosis for the return of bladder function (Penderis, 2008). Similarly, there is evidence to suggest that spinal cord injuries at C2–C4 are especially likely to be associated with the failure of respiration (Beal et al, 2001).
Extensive spinal cord lesions, especially those extending over two vertebrae, negatively influence prognosis as well (Tartarelli et al, 2005; Penderis, 2008).
Progressive myelomalacia is commonly reported with intervertebral disc extrusion, but progressive ascending and/or descending haemorrhagic necrosis of the spinal cord following acute, severe (especially thoracolumbar) spinal cord injury (Griffiths, 1972) can occur following vertebral fractures or luxations as well. The onset of the progressive myelomalacia can vary from less than 48 hours to 5 days after spinal cord injury (Castel et al, 2017). The non-specific clinical signs are present in 59% of affected dogs and can include diffuse hyperaesthesia, hypothermia, and/or hyperthermia (Castel et al, 2017). Progressive reflex loss of the pelvic limbs, loss of anal tone and urinary or faecal incontinence are presentations typical for descending progressive myelomalacia. Ascending progressive myelomalacia presents with more cranial cut-off cutaneous trunci reflex; sensorimotor reflex loss on the thoracic limbs and subsequent tetraparesis or paralysis; development of Horner's syndrome, or difficulties with ventilation (Jeffery, 2010; Castel et al, 2017). The reported occurrence of progressive myelomalacia ranges between 10 and 24,9% of dogs presenting with complete sensorimotor loss from spinal cord injuries (paraplegic with loss of pain perception in pelvic limbs and tail), following intervertebral disc extrusion (Olby et al, 2003; 2016; Aikawa et al, 2012; Fenn et al, 2020). A difference between breeds has been reported, with French Bulldogs (with loss of nociception at the time of presentation) demonstrating a prevalence of progressive myelomalacia as high as 33% (Aikawa et al, 2014). Progressive myelomalacia has also been reported to be more prevalent at the lumbar intumescence (L4–S3 spinal cord segments) (Castel et al, 2017; Shaw et al, 2017).
Although young animals (<12 months) are more prone to vertebral fractures and luxations (Bali et al, 2009), their prognosis for recovery is better given the high degree of plasticity in the nervous system in juvenile dogs (Olby et al, 2003). Prognosis can be influenced by the size of the patient (especially in dogs). Management of recumbent large breed dogs is arduous and can be a deciding factor when considering euthanasia (Cauzinille and Kornegay, 1996).
From the data extrapolated for other causes of spinal cord injuries (such as intervertebral disc extrusions or fibrocartilaginous embolism), patients suffering from vertebral fractures and luxations have a worse prognosis if:
- They have lack of nociception, especially for longer than 48 hours (Olby et al, 2003; Penderis, 2008)
- The areas of spinal cord intumescences are affected (vertebrae C4–T3 and L3–L5) (Jeffery, 2010; Penderis, 2008)
- More than two vertebrae are affected (Penderis, 2008)
- They are showing signs of progressive myelomalacia (Castel et al, 2017)
- They are older or a large/giant breed (Olby et al, 2003; Bali et al, 2009)
- There is a concurrent historical disease or injury (Penderis, 2008).
What is the best imaging modality to obtain vertebral fracture or luxation diagnosis?
Diagnostic imaging of the vertebral column and spinal cord plays a key role in the definitive diagnosis of vertebral fractures or luxations. Depending on the diagnostic imaging modality, information on the number, location and type of injuries can be obtained as well as an individual treatment plan (Jeffery, 2010). Imaging techniques include radiography, fluoroscopy, computed tomography (CT) and magnetic resonance imaging (MRI) (Bagley, 2000; Olby et al, 2003; Jeffery, 2010).
Radiographs provide an inexpensive and rapid means for initial evaluation of the vertebral column following trauma, but they have limitations. Sedation causing muscle relaxation and manipulation, which could potentially lead to iatrogenic trauma to the spinal cord, is usually required to achieve accurate positioning in the pursuit of obtaining diagnostic quality radiographs of the spine (Lewis, 1974; Dennis, 1987; Sande, 1992; Bagley, 2000). Thoracic and abdominal radiographs should be acquired as well, to identify additional soft tissue injuries (Turner, 1987; Bruce et al, 2008).
Multiple injuries to the vertebral column often occur (Bali et al, 2009; Jeffery, 2010). Therefore, radiographs of the whole vertebral column (from the first cervical to the coccygeal vertebrae) should be evaluated for evidence of fractures, luxations and subluxations. The presence of displaced fracture fragments is important to note, as this indicates that compression of the spinal cord in this location is likely. The radiographs should be evaluated for evidence of instability, based on the most used three-compartment or three-column model (Figure 12). The McAfee or Magerl classification systems, used in human medicine, also take into consideration the location of fracture lines in reference to tension, compression forces and spinal cord impingement by bone fragments or disc material. In the case of suspected atlantoaxial instability, if a subluxation is not visible on lateral radiographs, the cervical spine can be flexed carefully, while fluoroscoping, to identify instability. Fluoroscopic monitoring prevents the failure of the atlantoaxial junction during flexion (Jeffery, 2010; Loughin and Marino, 2016). If fluoroscopy is unavailable, MRI and CT are useful techniques to identify the extent of spinal cord injury, without a need for flexion of the head, as well as to allow for surgical planning (Figures 6 and 7).
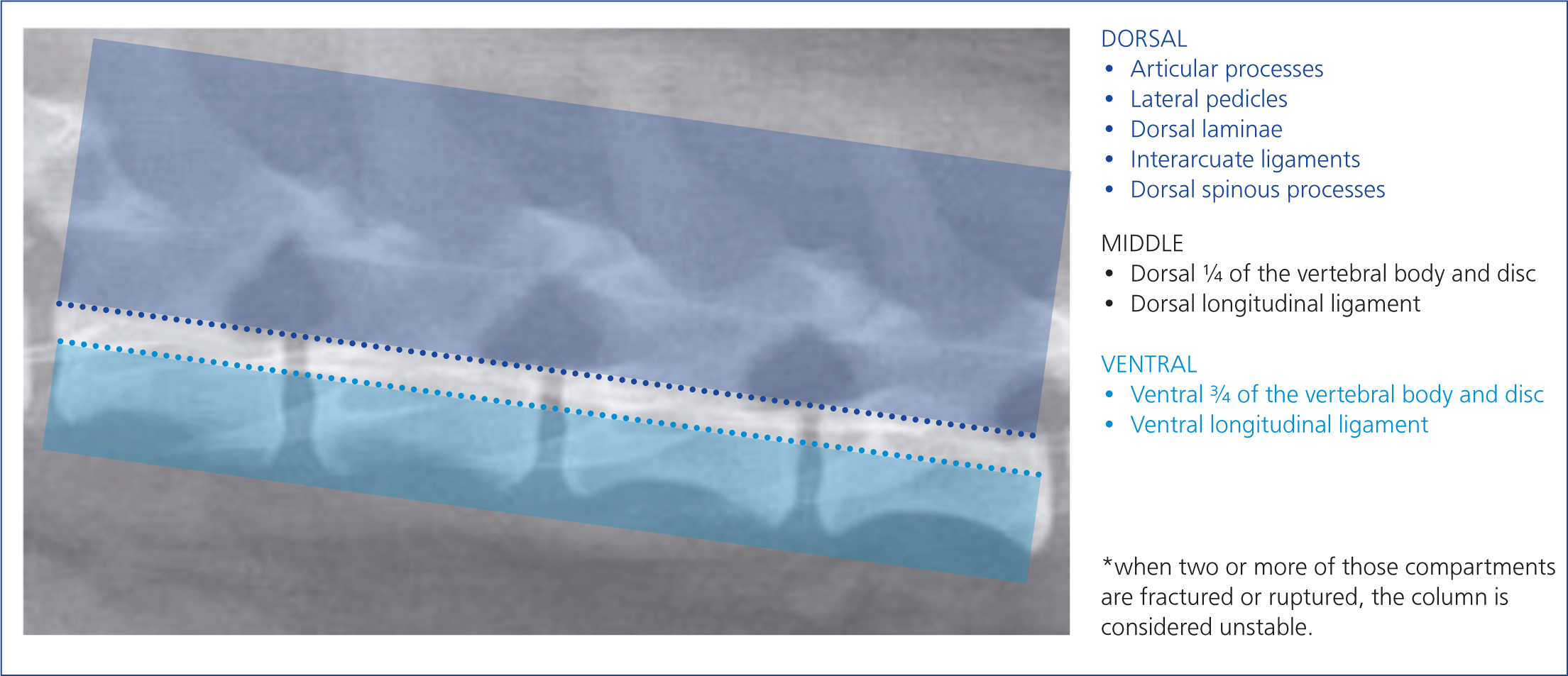
Sadly, radiography has only moderate sensitivity for fractures (72%) and subluxations (77.5%) and cannot be used to reliably rule out potentially unstable vertebral column lesions in canine and feline trauma (Figure 13) (Kinns et al, 2006).
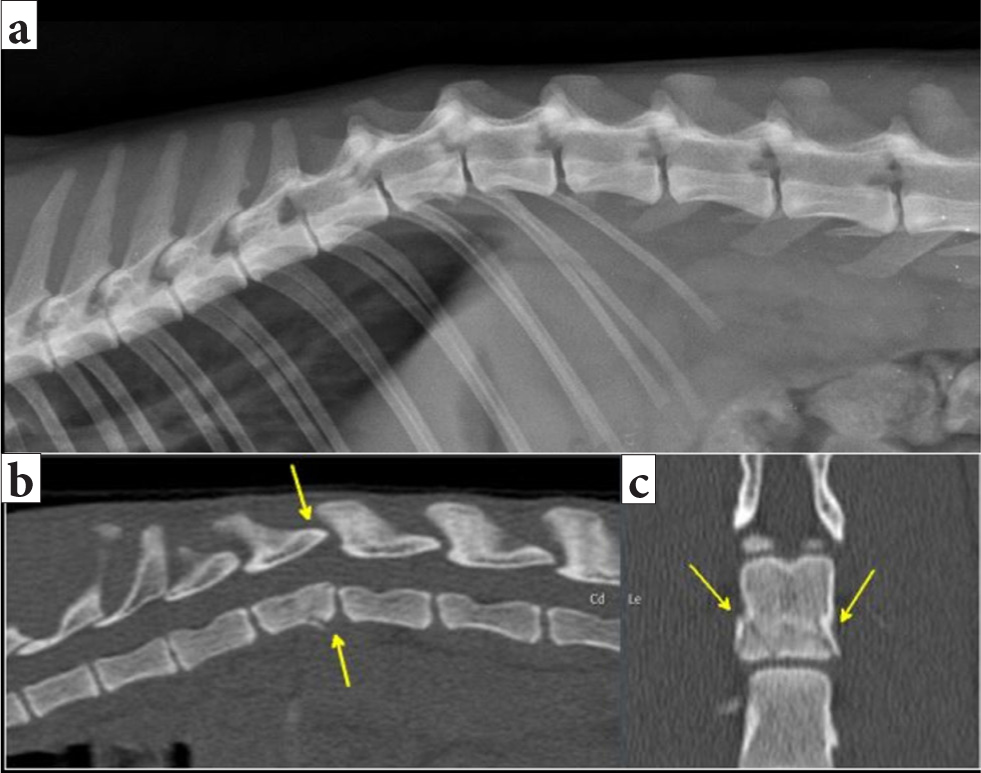
Myelography is not recommended, on account of the possibility of it causing transient deterioration in neurological signs, secondary to chemical myelitis, and other potential side effects such as seizures. Additional injury during positioning to obtain orthogonal views can occur as well (Jeffery, 2010; Sande, 1992).
Further evaluation of the injured vertebral column and spinal cord following trauma requires advanced imaging (CT and MRI). This is vital for surgical planning (Hettlich et al, 2010).
Cross-sectional imaging techniques (CT and MRI) overcome many of the limitations of survey radiography and myelography. The main advantages, besides their accuracy (Griffen et al, 2003; Mann et al, 2003; Sundgren and Flanders, 2012), are that they allow multiplanar evaluation and avoid the need for extensive patient manipulation. The modality of choice to assess for lesions in the bone is CT, with a reported sensitivity to vertebral fractures and luxations of up to 100% (Griffen et al, 2003; Mann et al, 2003) (Figure 9).
An MRI is the modality of choice for diagnosing spinal cord injury, as it assesses intervertebral discs and ligaments and can detect any vascular or soft tissue injuries. The only method that can differentiate spinal cord haemorrhage from oedema, which might be of prognostic significance and may influence the treatment, is MRI (Sundgren and Flanders, 2012). A lack of change in signal intensity in the spinal cord following injury is generally associated with a good outcome (Takahashi et al, 1993; Ito et al, 2005). However, it has been reported that in spinal cord injuries caused by intervertebral disc herniations and fibrocartilaginous embolism, dogs demonstrating an extensive intramedullary T2 weighted hyperintensity have worse outcomes (Ito et al, 2005). This can likely be applied to vertebral fractures and luxations as well (Figure 14).

An MRI may be able to detect the presence of fractured vertebrae aided by associated soft tissue changes, such as ruptured ligaments or changes in epaxial muscles (Johnson et al, 2012). However, it is not able to replace CT for the complete evaluation of the traumatised spine and documentation of fracture morphology (Johnson et al, 2012; Gallastegui et al, 2019).
Conclusions
Animals with vertebral fractures and luxations require a complex approach with prompt initial triage, including thorough clinical and neurological examination, with management aiming to maintain blood pressure and oxygenation. Together with imminent immobilisation this allows for stressless diagnostic imaging, ideally with CT scans. Clinical presentation, especially presence or absence of nociception, localisation, as well as the extent and character of the injuries, determine the prognosis.
KEY POINTS
- Initial assessment (airways-breathing-circulation), physical and neurological examination, and immediate immobilisation are all vital for successful management of animals with vertebral fractures and luxations.
- Providing sufficient blood flow and oxygenation to the spinal cord is essential.
- Appropriate analgesia is required for patients with vertebral fractures or luxations after immobilisation.
- The most sensitive and specific diagnostic modality for vertebral fracture and luxation is computed tomography.