In recent years, a growing body of evidence has started to elucidate the importance of the gastrointestinal microbiome in a variety of interactions with the host. It is now thought to have a number of significant impacts on a host's health. It has also been directly implicated in the pathogeneses of both gastrointestinal and systemic disease. Much of this research has been done in humans and rodents, accelerated by advances in the technologies available to analyse the microbiome, but an increasing number of studies are now being done on companion animals. This article explores the current understanding of the role of the gastrointestinal microbiome both in health and disease, as well as the role that dysbiosis may play in behaviour and disease states, such as gastrointestinal conditions, obesity and neurological diseases. It also briefly considers ways in which the gastrointestinal microbiome may be theraputically manipulated for companion animals. Box 1 outlines definitions of key terms used in this article.
Box 1.Key definitions usedMicrobiome:The genetic make-up of the whole of the microbiota. The genes from all of the bacteria, fungi and viruses that inhabit the intestinal tract (Valdes, 2018).Microbiota:The collection of all living microorganisms (bacteria, fungi, protozoa, viruses) inhabiting the gastrointestinal tract (Suchodolski, 2016).Intestinal dysbiosis:An alteration or imbalance in the intestinal microbiota composition and/or diversity (Suchodolski, 2016).Diversity:A measure of how many different species and, dependent on the diversity indices, how evenly distributed they are in the community (Valdes, 2018).Germ-free animals:Animals that have no microorganisms living in or on them (Valdes, 2018).Microbiota–gut–brain axis:The constant bidirectional communication between the gastrointestinal tract and the brain, with the gut microbiota playing a key role in this communication (Purina Institute 2019a).Prebiotics:Carbohydrate sources that are not digestible for the body, but which are specifically fermented by colonic bacteria to generate end-products such as short chain fatty acids, that provide essential nutrients for the enteric epithelium and have multiple other potential benefits in and outside of the gastrointestinal tract (Valdes, 2018).Probiotic:Live microorganisms that, when administered in adequate amounts, confer a health benefit to the host (World Health Organization, 2006).From World Health Organization, 2006; Suchodolski, 2016; Valdes, 2018; Purina Institute, 2019a
Methods of analysis of the gastrointestinal microbiome
Until relatively recently, traditional bacterial culture was commonly used to identify bacteria present in the gastrointestinal tract, but it is now recognised that the vast majority of intestinal bacteria cannot be cultured using standard plating techniques (Suchodolski, 2016). This means that culture alone has significant limitations and is not optimal for in-depth characterisation of an environment as complex as the mammalian gastrointestinal tract (Suchodolski, 2016). Faecal microbiota composition is often used to reflect the composition within the gastrointestinal tract, but this does not allow examination of any potential variations acids, which have a number of potentially beneficial roles in the in composition or metabolic activity in different areas of the gastrointestinal tract, and it also does not allow for consideration of other aspects, such as products of bacterial metabolism. Advances in technology, particularly molecular tools, are starting to enable identification of a greater number of the intestinal microbes, as well as providing information on the functionality of some of these species (Suchodolski, 2016). Molecular methods, mostly targeting the 16S ribosome genes (16S rRNA), are now the recognised standard for identification of bacterial microbiota. Molecular methods, targeted at the 16S rRNA gene, are being increasingly used for bacterial microbiota identification (Suchodolski, 2016)
16S ribosome genes
- The 16S rRNA are a universally conserved bacterial gene region, with hypervariable sequences
- Amplification and sequencing of this gene region enables the identification of microbial organisms
- This can be followed by statistical analysis of microbial identity and diversity, based on sequence similarity and comparisons referencing microbial genomic databasesSeveral techniques are available, including fluorescence in situ hybridisation (FISH), quantitative real-time PCR (qPCR), next-generation sequencing and metagenomics (shotgun sequencing of genomic DNA) (Suchodolski, 2016), although their use is currently still confined to the research setting. These techniques, used alone or in conjunction with one another, have enabled a more detailed understanding of the gastrointestinal microbiome. One study was able to demonstrate substantial differences in the microbiome in different segments of the gut in dogs. Lactobacillus was found to be distributed uniformly along the gastrointestinal tract of the dogs, but the relative abundances of Enterobacteriales, Fusobacteriales and Clostridiales varied along its length, with different species predominating in different areas of the intestinal tract (Suchodolski et al, 2008) (Figure 1).
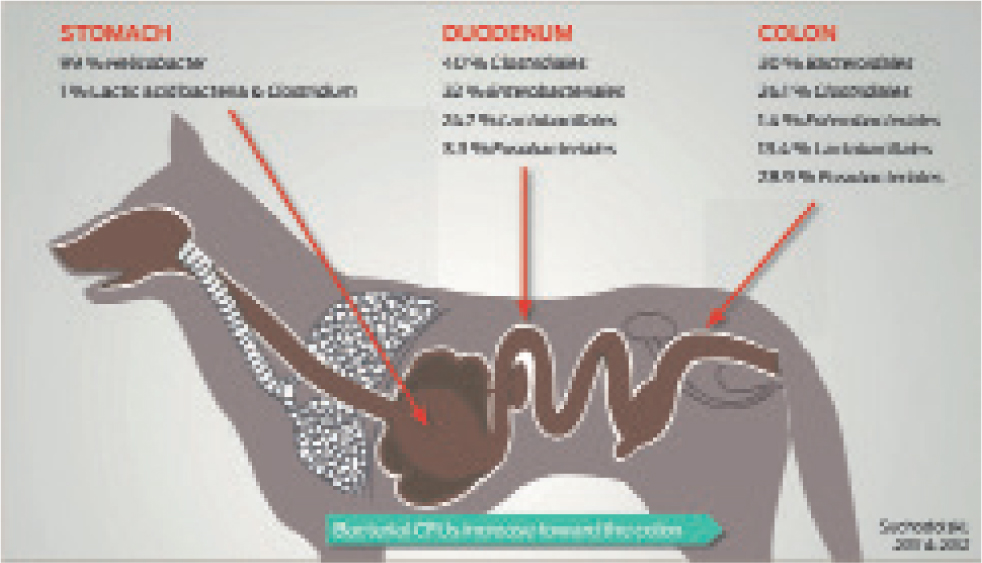
What roles does the intestinal microbiota have?
Each section of the gut has unique characteristics and different functions, such as nutrient digestion in the small intestine or water absorption in the colon. Different types and different quantities of bacteria have now been found to populate the various sections of the gut. The colon contains the greatest number and variety of bacteria, as intestinal transit time is reduced here, so bacteria have more time to flourish. There are an estimated 100 trillion microbial cells in the gastrointestinal tract, which is tenfold more than the number of host cells (Suchodolski, 2016). They have a number of roles locally within the gastrointestinal tract (Table 1), along with many additional beneficial effects that can reach beyond the tract (Suchodolski, 2017).
Table 1. Some key roles of the gastrointestinal microbiota
Immune system regulation | Direct contact between microbes and the immune system (for example, dendritic cells) |
Contact via microbiota-derived metabolites (such as secondary bile acids, which can be immunomodulatory and can strengthen intestinal barrier function) | |
Defence against enteropathogens | Creation of a physical barrier to pathogen colonisation, competition for nutrients and production of antimicrobial substances |
Influences on the immune system | |
Nutritional benefits | Short chain fatty acids are one of the most important gut microbial products, affecting a range of host processes, including energy utilisation, host-microbe signalling and control of colonic pH, with consequent effects on microbiota composition, gut motility and epithelial cell proliferation |
Development of gastrointestinal structure | Germ-free mice have an altered epithelial architecture |
The gastrointestinal microbiota provide a number of nutritional benefits (Suchodolski, 2017; Valdes, 2018). Intestinal microflora synthesise and supply vitamins to the host, particularly B vitamins including folate and cobalamin (LeBlanc et al, 2013). Complex carbohydrates, such as inulin from chicory, are fermented by bacteria in the large intestine to produce short chain fatty gastrointestinal tract, including acting as an energy source for the host; intestinal motility regulation and direct anti-inflammatory properties through expansion of immunoregulatory lymphocytes (Suchodolski, 2017) (Figure 2). Butyrate is the main energy source for colonocytes and is essential for epithelial cells to consume large amounts of oxygen, generating a state of hypoxia that maintains oxygen balance in the gut and preventing dysbiosis (Valdes, 2018). Acetate, the most abundant fatty acid, is an important metabolite for the growth of other bacteria and is also transported into peripheral tissues, where it plays a role in cholesterol metabolism and lipogenesis (Valdes, 2018). Propionate has been found to regulate gluconeogenesis and also influences satiety signalling in humans (Valdes, 2018). Randomised controlled trials in humans have shown that higher production of short chain fatty acids correlates with lower diet-induced obesity and reduced insulin resistance. In mice, some of these short chain fatty acids seem to reduce appetite and food intake (Valdes, 2018).
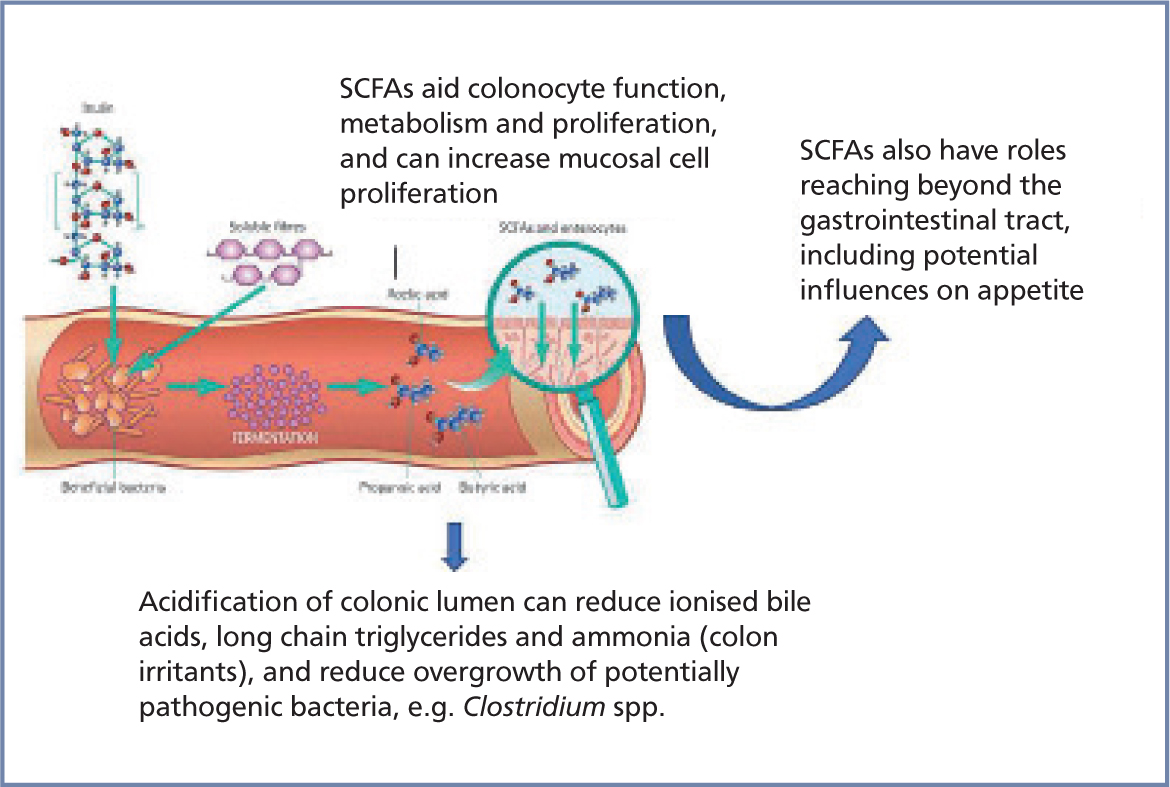
Gut microbiota also modify the metabolism of cholesterol and bile salts. In fact, gastrointestinal bacteria are solely responsible for the conversion of primary bile acids to secondary bile acids (Suchodolski, 2016), which is one example of how important they are to the physiology of dogs, cats and humans. These secondary bile acids act as signalling molecules and metabolic regulators to influence important host pathways (Valdes, 2018). They are immunomodulatory and help to strengthen intestinal barrier function. Changes in intestinal bile acid metabolism are implicated as an important factor in intestinal inflammation in human patients with inflammatory bowel disease (Lin et al, 2016). Unconjugated bile acids are toxic to epithelial cells, which may result in increased intestinal permeability, where altered bile acid profiles lead to changes in fat absorption from the small intestine (Suchodolski, 2017). There is emerging evidence that altered bile acid metabolism leads to bile acid malabsorption, a frequent cause of diarrhoea in humans (Lin et al, 2016). It has also been shown that therapeutic correction of altered bile acid concentrations can lead to a reduction of intestinal inflammation (Lin et al, 2016). Significant correlation between an increased dysbiosis index and bile acid dysmetabolism has also been documented in dogs with chronic enteropathies (Suchodolski, 2017). Intestinal bile acid metabolism is now also becoming of increased interest within the veterinary field.
In addition to aiding digestion and providing energy to the host, the gut microbiome plays key roles in protection from enteropathogens by inhibition, displacement and competition; support of the intestinal mucosal integrity and multiple roles in immunity and inflammation (Rooks and Garrett, 2016). The gut microbiome can influence both the systemic and gastrointestinal immune system of the host. A ‘healthy’ microbiome provides crucial molecular cues through microbial surface antigens and metabolites, which are essential for the maturation of immune tissues and for the fine tuning of immune responses (Rooks and Garrett, 2016). These interactions begin at birth and continue throughout life.
What constitutes a healthy microbiota?
A balanced microbial ecosystem is of crucial importance for its host's health (Suchodolski, 2016). Every individual has a very different microbiome, differing both in the balance and the species and strains of bacteria within the gastrointestinal tract (Suchodolski, 2016). However, marked differences in the microbiome present in a healthy animal as opposed to a diseased animal. Over time, certain disease phenotypes demonstrate specific patterns of microbiome shifts (Honneffer et al, 2014). For example, members of some Clostridium clusters are more consistently depleted in humans and dogs with inflammatory bowel disease and acute colitis, suggesting that these groups are highly important in maintaining intestinal homeostasis (Honneffer et al, 2014). This research is still in its early stages, and at this point in time, it is still do not know what constitutes a healthy microbiome. Further information about microbial composition and their functions, including their metabolic activity and immune interactions, is needed to define what is ‘healthy’ or ‘unhealthy’, as well as the implications of this.
What factors influence development of a healthy microbiota?
Early microbiome development
Studies in humans have demonstrated that neonatal acquisition of the intestinal microbiome depends on the organisms encountered in the first days of life, from the mother and the environment. The microbiome then undergoes a developmental maturation process, guided by interactions with the host in which its composition and functional capacity mature (Barko et al, 2018). There are limited studies in companion animals, but a study in one group of kittens has shown that the early faecal microbiome demonstrates a large amount of interindividual variation and that intraindividual diversity and composition stability increase with age, which is the same in humans. The early microbiome is thought to have roles in enabling adaptation of the host to increasingly complex nutrients and in directing development of the immune system, gut epithelium and brain (Barko et al, 2018). Its active participation in developing host physiology means that if microbiome balance is disrupted, it could have negative health consequences (Barko et al, 2018).
Genetic and environmental factors
Both genetics and environmental factors can influence microbiota balance (Figure 3). The influence of host genetics on the composition of the gut microbiome is still unclear, with some studies in humans showing the intestinal microbiome of relatives to be more similar in composition than non-related individuals. However, another large-scale study that included some pairs of twins found that the gut microbiome was no more similar in relatives as opposed to unrelated individuals (Barko et al, 2018). Diet and environment are thought to be particularly important influencers of the gut microbiome, starting from a young age (Vester et al, 2009; Hooda et al, 2013; Barko et al, 2018). In their study, Vester et al (2009) demonstrated that diet is a key influencer of gut microbiome in young cats. The kittens were separated into two groups and fed either a moderate or high protein diet after weaning. Microbiome analysis showed that the kittens showed similarities to others in their litter at 8 weeks of age, which potentially supports the role of genetics in microbiome balance. However, the effects of kinship were decreased at 12 weeks compared to 8 weeks of age, and diet appeared the primary factor affecting the similarities and differences found between the cats (Vester et al, 2009). In another study of kittens, variations in the dietary protein to carbohydrate ratio were shown to impact the faecal microbiome (Hooda et al, 2013). This study also demonstrated variations in circulating hormones and metabolites, such as triglycerides, blood glucose, cholesterol and leptin, which correlated with the faecal microbe populations. These suggested diet-induced changes in the microbiome may be important in terms of satiety and host metabolism. Fermentable carbohydrates can also change the gut's microbial populations, and other studies have shown that the addition of dietary fibre in dogs can affect the overall composition of the gut microbiome (Middelbos, 2008).
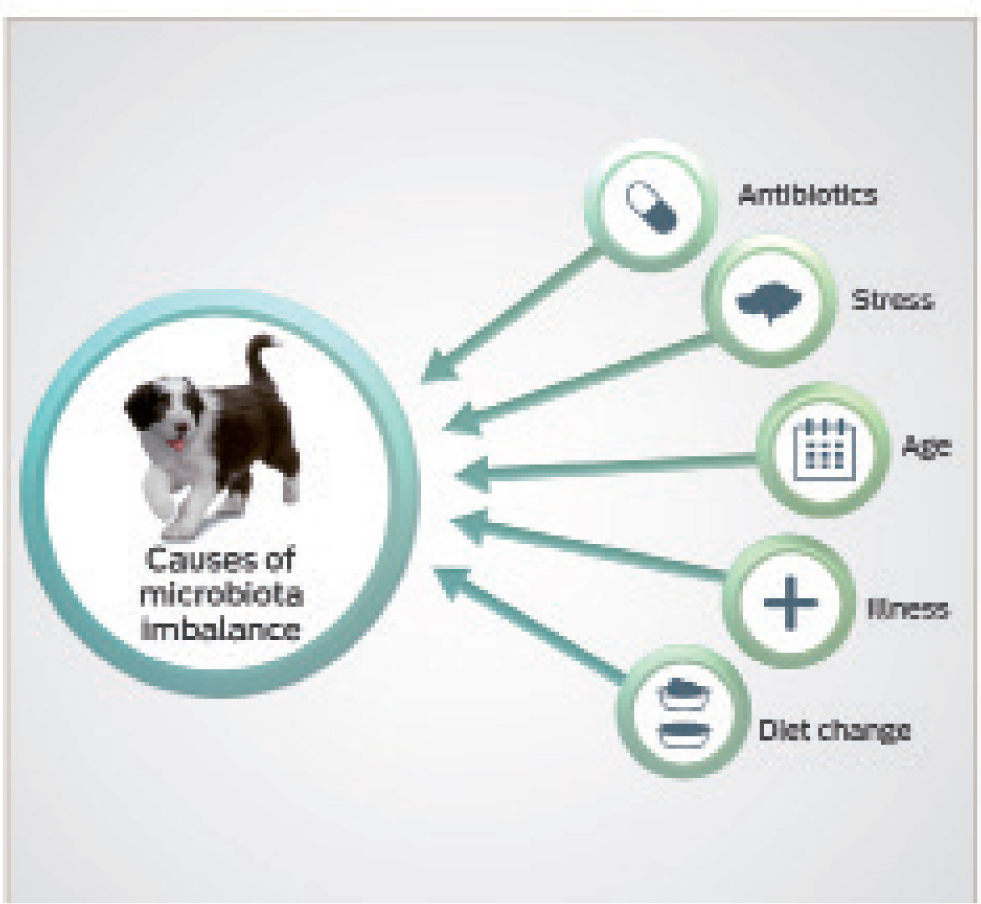
Medical interventions
Medical interventions, both early and later in life, may also influence microbiome stability. In humans, for example, ciprofloxacin administration has been found to rapidly and significantly reduce the diversity and richness of the faecal microbiome within 4 days of starting the medication, only partially recovering to its original composition after discontinuation (Dethlefsen and Relman, 2011). In healthy dogs, macrolide administration (with tylosin) has been shown to induce a decreased diversity in faecal microbiome, although this was not associated with any short-term clinical effects of gastrointestinal disease in dogs (Suchodolski et al, 2009). A persistent long-term reduction in diversity and richness was seen in 40% of dogs (Suchodolski et al, 2009). In another study of healthy pet dogs, a 14-day administration of metronidazole caused a dysbiosis in the study population and led to development of diarrhoea in 9 out of 16 dogs, resolved only after the end of the administration period (Olson et al, 2015). In comparison, none of the control population (who did not receive metronidazole) developed diarrhoea. Interestingly, metronidazole led to a decrease in bacterial groups constituting major abundant groups in the gastrointestinal tract of healthy dogs, which are thought to be important for maintaining intestinal health (Olson et al, 2015).
Antibiotic perturbation may cause a shift to an alternative stable state, the full consequences of which remain unknown (Dethlefsen and Relman, 2011), but this is perhaps something we should start to consider more as clinicians, when administering antimicrobial agents to companion animals. In addition to antibiotics, other medications, including omeprazole, also appear to impact canine faecal microbiome stability (Barko et al, 2018). Further research is warranted to better understand the full impact of medical interventions on microbial stability.
The association between dysbiosis and disease states
A dysbiotic microbiome may result in functional or immunological impacts on the host, which can be deleterious. For example, they can be a result of the production of toxins, or reduction in anti-inflammatory metabolites (Suchodolski, 2016). The extent of these changes will depend on the magnitude and the pattern of dysbiosis (which bacterial groups are altered) and the location of dysbiosis (small intestine vs large intestine) (Suchodolski, 2016). The diversity of the intestinal microbiome is emerging as a critical determinant of host health. A loss of diversity has been associated with a variety of gastrointestinal and systemic diseases in humans and other mammals (Barko et al, 2018). However, it can be difficult to correlate dysbiosis with the clinical picture, as it can be present in patients with no symptomatic disease. Dysbiosis and its contribution to disease seems to vary between different patients.
Gastrointestinal disease
Dysbiosis is often associated with acute and chronic gastrointestinal disorders (Suchodolski, 2016; 2017), although in the majority of cases, it is still unknown whether dysbiosis is the cause or the result of disease. Dysbiosis has been described in dogs with gastrointestinal diseases, including inflammatory bowel disease and acute diarrhoea, in cats with chronic enteropathies and in both dogs and cats infected with Giardia duodenalis (Honneffer et al, 2014; Suchodolski, 2016). It has also been associated with intestinal stasis and other motility disorders, decreased gastric output and exocrine pancreatic insufficiency (Suchodolski, 2016). However, it is not known whether dysbiosis patterns differ between different gastrointestinal diseases, for example, in different forms of chronic enteropathies including food-responsive enteropathy, antibiotic-responsive enteropathy and inflammatory bowel disease (Suchodolski, 2016). Long-term follow-up studies are also needed to examine whether changes in microbiota can revert with clinical remission. Some studies have suggested that the microbiota remains altered because of an underlying disease or residual histological inflammation present in the intestine (Rossi et al, 2014). Further studies are needed to correlate the long-term rate of clinical relapse in animals, with the dynamics of dysbiosis. Proper characterisation of dysbiosis is also desirable, to enable a better understanding of processes in animals with gastrointestinal disease and help guide treatment decisions (Suchodolski, 2016).
The impact of the therapeutics used also needs to be considered and further examined. The influence of antibacterial agents on dysbiosis is potentially very complex. Veterinarians may give antimicrobial agents to an animal with chronic diarrhoea and some patients may benefit from this. However in other individuals, it could induce or worsen any dysbiosis, potentially inducing worse diarrhoea. Dysbiosis can be induced by administration of antibiotics including tylosin and metronidazole, as well as resulting in clinical signs in itself (Suchodolski et al, 2009; Olson et al, 2015). Continued administration, during treatment of chronic gastrointestinal disease, could lead to the false impression on follow-up samples that the dysbiosis is persisting as a result of the disease, whereas the changes could actually be attributed to antibiotic treatment itself (Suchodolski, 2016). Hence, if antimicrobial agents are administered chronically, it is advised that serial evaluation of dysbiosis should be interpreted with care (Suchodolski, 2016).
Disease beyond the gastrointestinal tract
Dysbiosis also appears to play an important role in extraintestinal diseases including obesity, diabetes mellitus, atopic dermatitis and asthma (Craig, 2016; Barko et al, 2018). It also has roles in cognition and behaviour (Purina Institute, 2019c).
Obesity
There is significant evidence to support the link between obesity and certain forms of intestinal dysbiosis in humans, laboratory animals and pets (Barko et al, 2018). Obesity is a major problem in both human and veterinary medicine, so diagnostic and therapeutic tools to prevent and treat it are in demand, emphasising the need for further research in this area.
Most studies of overweight and obese people show a dysbiosis characterised by a lower diversity (Valdes, 2018). Germ-free mice that receive faecal microbes from obese humans have been observed to gain more weight than mice that receive microbes from humans with a healthy weight (Valdes, 2018). Additional studies of mice have suggested a direct causal link between the intestinal microbiome and increased adiposity (Barko et al, 2018). Gut microbiota dysbiosis may promote diet-induced obesity and metabolic complications, resulting from immune dysregulation, microbe-induced changes in host glucose and lipid metabolism, altered gut hormone regulation and stimulation of chronic low grade inflammation, which could further result in insulin resistance (Barko et al, 2018; Valdes, 2018). In a pilot study looking at the gastrointestinal microbiome of obese cats, moderately overweight and obese cats were found to have a different microbiome and in particular, lower proportions of Bacteroidetes, compared to healthy cats (Kieler et al, 2016). This is similar to previous studies of humans, which found that in a group of mothers and twins, obese individuals had lower proportions of faecal Bacteroidetes than their lean relatives (Barko et al, 2018). However, a study comparing lean and obese pet dogs found no marked shifts in the microbiome associated with obesity. Additional studies are needed to clarify the role of the microbiome in obesity (Barko et al, 2018).
Atopic dermatitis
Most research into the role of the intestinal microbiota in atopic dermatitis has been done in humans (Craig, 2016). Impairment of the intestinal mucosal barrier appears to be involved in the pathogenesis of atopic dermatitis in humans. This is a risk factor for canine food allergy, which is known to predispose a subset of dogs to this condition (Craig, 2016). Differences in the faecal microbiota have been demonstrated between human patients with atopic dermatitis and healthy control subjects. Systemic antibiotic treatment has been reported to increase the risk in people, potentially resulting from changes in intestinal microbiota (Craig, 2016). Given the observations that an impaired intestinal microbiota may be involved in the pathogenesis of human atopic disease, as well as the parallels seen between humans and dogs, it has been suggested that manipulation of the canine intestinal microbiota could be considered, as a way to help manage atopy (Craig, 2016). In one double-blind, placebo-controlled study in dogs diagnosed with canine atopic dermatitis, oral administration of a heat-killed faecalis FK-23 preparation, resulted in significant improvements in the canine atopic dermatitis extent and severity index. The study's authors concluded that this provided a small but measurable benefit, in reducing clinical signs of atopic dogs when used as an adjunct treatment (Osumi et al, 2019). There is currently insufficient evidence to draw any clear conclusions, but further studies are warranted in this area to allow future recommendations to be made.
Cognition and the ‘microbiota–gut–brain axis’
The term ‘gut–brain axis’ refers to the constant bidirectional communication between the gastrointestinal tract and the brain (Purina Institute, 2019c). The gut microbiota are emerging as a key node in the communication between gut and brain, leading to the coining of a new term ‘microbiota–gut–brain axis’ (Purina Institute, 2019c).
There are several mechanisms by which the gut microbiota may communicate with the brain and central nervous system (Purina Institute, 2019c):
- Enteric nervous system: composed of millions of neurons extending along the length of the gastrointestinal tract, it communicates changes within the tract to the brain, via the vagus nerve
- Sympathetic nervous system: the release of adrenaline and noradrenaline from the brain and adrenal glands leads to behavioural responses, which can alter gut motility
- Hypothalamic–pituitary–adrenal axis: mediates cortisol secretion, which influences the immediate stress response. The gut microbiota may affect the development of the neural systems governing the endocrine response to stress
- Immune signalling: the gut microbiota may affect immune cells in the gut mucosa that release cytokines, which are important for host responses to inflammation and infection, which modify enteric nervous systems' sensory neuron activity
- Gut bacterial metabolites: short chain fatty acids produced from fibre sources by the gut bacteria are particularly important metabolites, with possible influences on brain function. The gastrointestinal tract and its microbiome also contribute to the body's production of hormones and neurotransmitters, including dopamine and serotonin.
Perhaps the strongest evidence for the notion that gut microbiota can affect the brain comes from clinical practice in hepatology. Hepatic encephalopathy can occur in animals with end-stage liver disease and the effects on the animal's cognition can vary, from small changes in sleep pattern or a reduced attention span, to coma in severe cases. Although it is difficult to be sure of cause and effect, when enemas are given in acute disease, or when lactulose is administered as a laxative or antibiotics are given, particularly longer term, this can change gut microflora populations quite rapidly, resulting in a dramatic improvement in the cognition of the animal. This has been accepted within veterinary medicine for a number of years, but studies of the microbiota–gut–brain axis in a number of other contexts are now emerging.
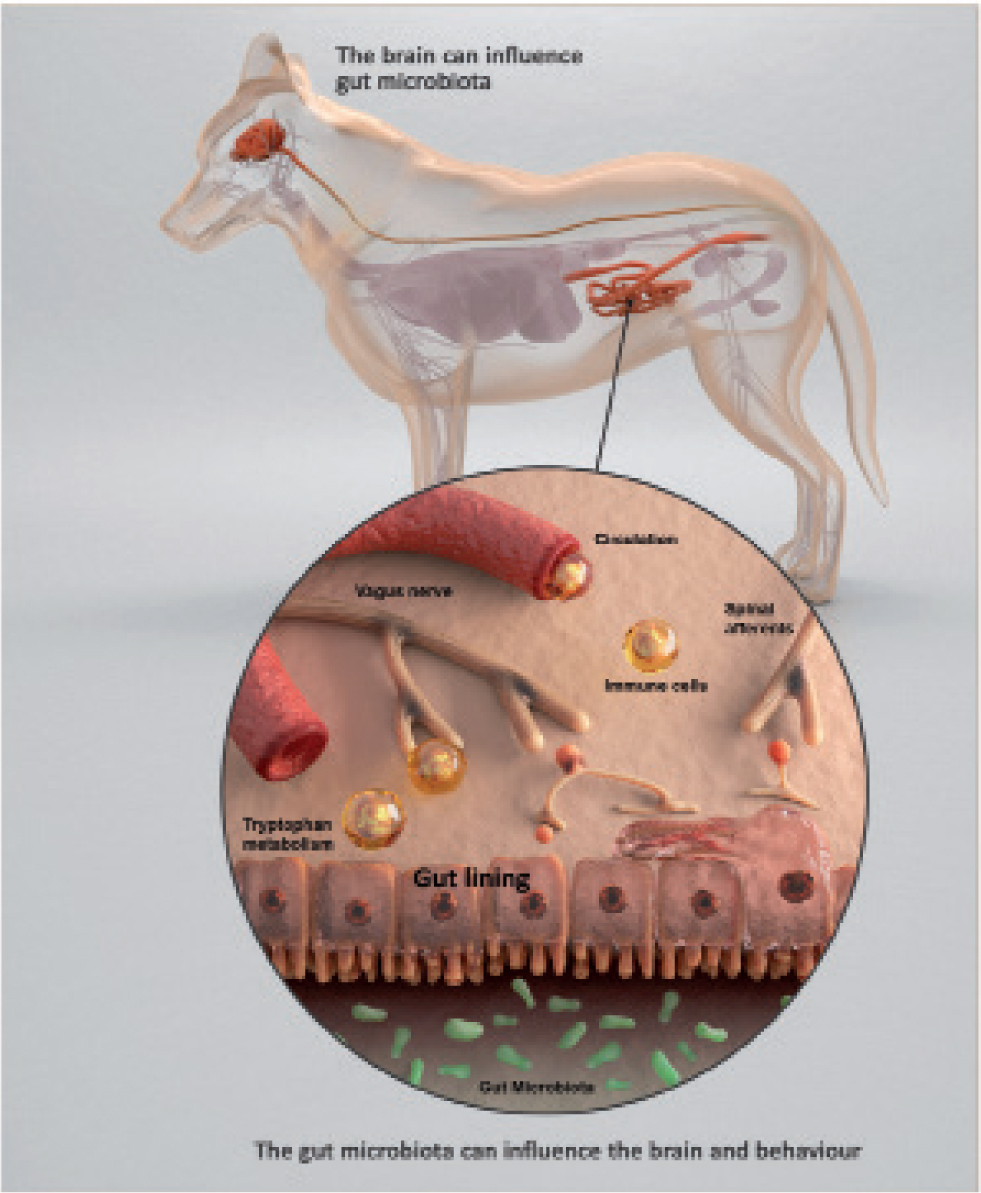
Studies in mouse models have now demonstrated that the gut microbiota can influence brain development programming, plasticity and the stress response (Sudo et al, 2004). Also, dysbiosis has increasingly been linked to impacts on cognition and behaviour (Collins et al, 2013), having been shown to be involved in the pathogenesis of neurological diseases (Gazzaniga and Gazzaniga, 2017). For example, bacterial analyses of faeces show that human patients with autism (Parracho et al, 2005), multiple sclerosis (Gazzaniga and Gazzaniga, 2017) and Parkinson's disease (Sochocka et al, 2019) all have disrupted microbiota, compared to healthy controls. Manipulation of the gut microbiota can directly influence anxious behaviours. The transfer of anxious behaviours has been seen in some murine studies when the gut microbiota are transferred (Collins et al, 2013), even in the absence of non-behavioural phenotypes, such as obesity, that are associated with anxiety (Bruce-Keller et al, 2015). In dogs, one placebo-controlled crossover study conducted in anxious Labrador retrievers, showed a reduction in anxious behaviours when a probiotic (Bifidobacterium longum BL999) was administered orally for 6 weeks (McGowan et al, 2018). This product is now commercially available for dogs in the USA. Some researchers are also hopeful that modulation of the gut microbiota has the potential to become a new treatment for Alzheimer's disease in humans (Sochocka et al, 2019). The potential for therapeutic options, targeted at altering the gut microbiota to impact on neurological disease and behaviour, is now a growing area of research in both human and veterinary medicine.
What can we currently do clinically to impact the gastrointestinal microbiome?
There are four main ways we can currently influence the microbiome in veterinary medicine. These are through antibiotics; diet and prebiotics; probiotics; and more novel therapeutic strategies such as faecal matter transplantation, although this is not currently widely available and tends to be reserved for only a small number of patients. Since each individual has such a unique and highly varied microbiome, companion animals may have very different clinical responses to each of these strategies.
The traditional approach of administering antimicrobial agents has increasing cause for concern, because of antimicrobial resistance and the negative impacts it appears to have in inducing major shifts in microbiota, sometimes causing diarrhoea as an unwanted side effect. At the moment, we have no way to block the effect of antimicrobial agents on dysbiosis. Antibiotic-induced dysbiosis has the potential to trigger longer term problems. In humans, antibiotic-induced dysbiosis in early childhood has been identified as one of the main risk factors in the development of allergies, obesity and inflammatory bowel disease. We should therefore start to consider using antibiotics more cautiously. However, it is important to highlight that in some patients antimicrobial agents may play a beneficial role in controlling diarrhoea. It can be challenging to identify which individuals may benefit from this approach and which may not, but given the potentially negative impacts of antimicrobial agents on the intestinal microbiome, this suggests that other approaches to management of gastrointestinal disease, such as nutritional management, prebiotics and/or probiotics, should be trialled first.
Nutrition can have a profound impact on the gastrointestinal microbiome, particularly in the form of fermentable carbohydrates that can change the gut's microbial populations and affect the overall composition of the gut microbiome (Middelbos, 2008). A number of veterinary diets containing prebiotics are now available in the UK, for both cats and dogs, found both within some maintenance diets for healthy pets and some diets tailored towards support in gastrointestinal disease. There are also many veterinary-authorised probiotic products, although there is great variability in the quality and potential efficacy of these products. Clinicians are therefore encouraged to assess the evidence for the particular strain and preparation of probiotic being marketed, before selecting it for use. Further discussion of probiotics is beyond the scope of this article, but several excellent reviews exist on the subject (Chandler, 2014).
In addition to this, advising owners on how to help minimise stress in their animals, which may adversely impact microflora diversity and stability, for example, in incidences of environmental changes, may help support the gastrointestinal microbiome. Appropriate guidance to owners during any dietary changes, including a gradual diet transition and considering the use of gastrointestinal supportive products during this switch, may also help minimise changes in microbiome stability.
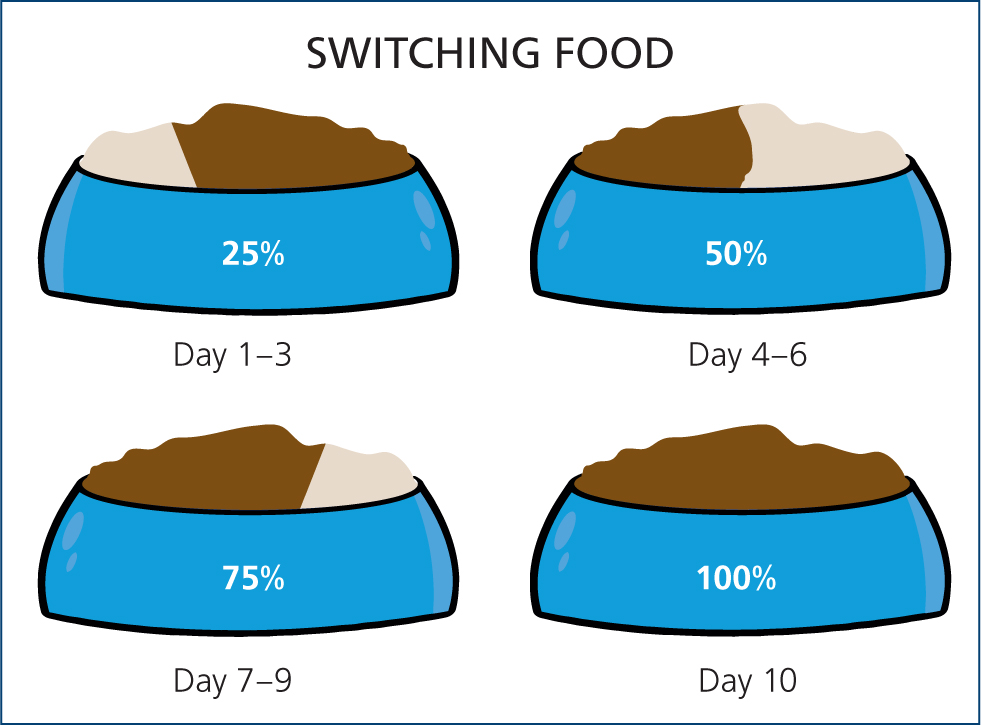
Conclusions
Knowledge of the gut microbiota is increasing rapidly, with over 10 000 papers published in recent years (Purina Institute 2019c). Fewer than 20% of intestinal bacteria can be cultivated using standard laboratory techniques (Suchodolski, 2016), but as technology advances, insights are being gained into the complexity of the intestinal microbiota and the important roles it may have in development and maintaining health. The role dysbiosis may play in the pathogeneses of disease, in both gastrointestinal disorders and systemic diseases, is becoming better understood. However, it is important to highlight that, at this stage, many roles of the microbiome in cats and dogs remain largely hypothesised, emphasising the need for further studies and research.
Many unanswered questions remain. Further understanding of dysbiosis, the factors that influence it and the potential clinical implications for companion animals is crucial, to help fully understand the therapeutic strategies currently used in veterinary practice, to help manage acute and chronic gastrointestinal disorders and dysbiosis, as well as how to influence the microbiome beneficially. This knowledge may enable us to identify potential diagnostic and therapeutic targets, in a variety of gastrointestinal and systemic conditions. Even at this time, there is substantial evidence to suggest we may need to carefully reconsider our use of antibacterial agents. Some of the conditions previously treated, or that are still treated, with antibacterial agents may not actually merit the use antibacterial agents as a first line approach and clinicians should be mindful that antibiotic administration, in itself, may induce dysbiosis.
Future studies will need to better elucidate the underlying mechanisms of interactions between the microbiome and host. They will also need to incorporate measurements of bacterial metabolites, such as faecal bile acids and short chain fatty acids, to assess microbiota function and to evaluate changes in the host's immune system. In-depth characterisation of a greater number of species and their potential roles is also needed. Clarification of the role the gastrointestinal microbiome may have in different disease states is required, to design therapeutic tools that may help the assessment and treatment of conditions associated with intestinal dysbiosis. At this stage, nutritional support in the form of prebiotics and supplements such as probiotics show great potential, although more controlled studies and evidence are needed, to progress what is now a rapidly developing and exciting field of work.
Many unanswered questions remain amd further understanding of the gastrointestinal microbiome, dysbiosis, the factors that influence it and their potential clinical implications for companion animals is crucial. This will help to identify future diagnostic and therapeutic targets in the miciobiome, for the benefit of animal welfare.
KEY POINTS
- The gastrointestinal microbiome is an incredibly complex ecosystem, the balance of which is of crucial importance for the host's health.
- Recent developments in technology have allowed researchers to better understand the microbiome make-up, its complexity, its interactions with the host and its roles in health and disease.
- The roles of the gastrointestinal microbiome include aiding in digestion, providing nutritional benefits to the host, immune regulation and defence from enteropathogens, as well as extra-intestinal roles
- Every individual has a unique microbiome, but marked differences can be seen between the microbiome of healthy animals compared to diseased animals.
- Imbalances or reductions in the diversity of the gastrointestinal microbiome (dysbiosis) have been directly implicated in the pathogenesis of diseases.
- There are four main ways clinicians can currently influence the microbiome in veterinary medicine: antibiotics; diet and prebiotics; probiotics and faecal matter transplantation. Clinicians should be aware that the use of antiobiotics has the ability to induce dysbiosis.
- Manipulation of the microbiome may enable us to create new therapeutic strategies, to manage conditions including enteropathies, obesity and atopic dermatitis.