Radiotherapy is a unique treatment modality that consists of the medical use of ionising radiation. It plays a major role in management of human cancer oncology, with approximately 50% of cancer patients receiving radiation therapy during their course of illness (Delaney et al, 2005). The use of radiotherapy in companion animal oncology has developed and expanded significantly over the past 20 years; it is currently considered a fundamental therapeutic tool (McEntee, 2004; 2006; Farrelly and McEntee, 2014; LaRue and Custis, 2014).
Ionising radiation can damage tumour cells both directly, or indirectly through formation of free radicals (Johns and Cunningham, 1969). The main target is DNA, although ionising radiation can also damage other important cellular structures such as the cytoplasmic membrane (Halperin et al, 2013; Hall and Giaccia, 2018). Tumour cells typically die following an unsuccessful attempt to divide, as a result of their damaged DNA, although exquisitely sensitive cells, such as lymphocytes, can die within hours via apoptosis. Some cells undergo senescence, where they remain alive but incapable of dividing (Ward, 1988; Harding et al, 2013).
There are multiple types of ionising radiation used for tumour treatment, including electromagnetic (photon based, such as X-rays or gamma rays) or particle (proton, electron or neutron based) radiation (Locher, 1936; Koehler and Preston, 1972). In veterinary medicine, therapeutic use of ionising radiation mostly involves electron and photon therapy because of the costs and limited availability of proton and neutron therapy. Each radiation type has specific radiobiological characteristics and dose distribution patterns, which can make them more or less desirable depending on the lesion type and location (Leaver and Alfred, 2004). Electron therapy, for example, is used primarily for superficial lesions, because it has a rapid dose fall off after a specific depth, thereby sparing normal underlying tissue. Photon therapy is preferred for deep-seated lesions, although it can also be used for superficial tumours, especially when electron therapy cannot be considered (e.g. if the area to be treated has uneven surfaces) (McEntee, 2006).
Radiotherapy treatment can be classified by means of administration, including teletherapy, brachytherapy, and systemic radioisotope therapy. Teletherapy, also known as external beam radiotherapy, is the most common form of radiotherapy used in veterinary medicine (Withrow et al, 2019).
External beam radiotherapy is further classified based on the energies used, which determine the depth of penetration by the beam (Halperin et al, 2013; Ettinger et al, 2016). Orthovoltage units use lower energies (150–500 kVp), which are associated wtih greater skin and bone toxicity. Megavoltage radiation (>1 MV) predominates in veterinary medicine because of its increasing availability and wide applicability in different tumours (Bentel, 1996; Moore, 2002; Leaver and Alfred, 2004; McEntee, 2004; 2006). This type of radiation has greater depth of penetration and spares the skin, allowing for improved treatment of deep-seated lesions. Megavoltage radiation has largely transitioned from cobalt-60 teletherapy machines (1.25 MV) to high energy (6–15 MV) linear accelerators (McEntee, 2004; 2006; LaRue and Custis, 2014). Unlike cobalt machines, which can only treat with photons, linear accelerators are capable of producing both photons and electrons. In addition, linear accelerators can treat using a broader range of megavoltage energies (Leaver and Alfred, 2004; LaRue and Custis, 2014) and can offer superior precision techniques by using advanced technologies including multileaf collimators, on-board imaging devices and advanced software. These technologies enable highly precise and accurate radiation treatments such as intensity modulated radiotherapy or stereotactic approaches (LaRue and Custis, 2014; Gieger and Nolan, 2017; Nolan and Gieger, 2019).
Table 1 presents different teletherapy treatment techniques. When using teletherapy, computer-based three-dimensional conformal protocols are preferred for most treatment sites, as these enable significantly improved dosing accuracy and sparing of the normal surrounding tissues. However, these clinical advances incur higher costs associated with the increased complexity of radiotherapy planning and the need for a computed tomography scan for treatment simulation (Figures 1–5) (Prado and Prado, 2004; Withrow et al, 2019). Manual radiotherapy plans are less expensive and less time consuming than computer plans, but their use is typically limited to single field or parallel opposed photon plans for tumours affecting the extremities, or for superficial lesions managed with electron treatments (McEntee, 2006) (Figures 6 and 7). New techniques of treatment planning and delivery have been developed that allow much more accurate dose-shaping to the tumour, with improved sparing of the surrounding tissues (Table 1) (Nolan and Dobson, 2018). This new era of radiation oncology has expanded dramatically in veterinary medicine in the last few years, with increasing availability in countries worldwide, including the UK (Nolan and Gieger, 2019; Rohrer et al, 2019).
Table 1. Summary of most common techniques in external beam radiotherapy
2D manual radiotherapy |
|
3D conformal radiotherapy |
|
Intensity modulated radiotherapy |
|
Volumetric arc therapy |
|
Stereotactic radiation therapy |
|
Imaging-guided radiotherapy |
|
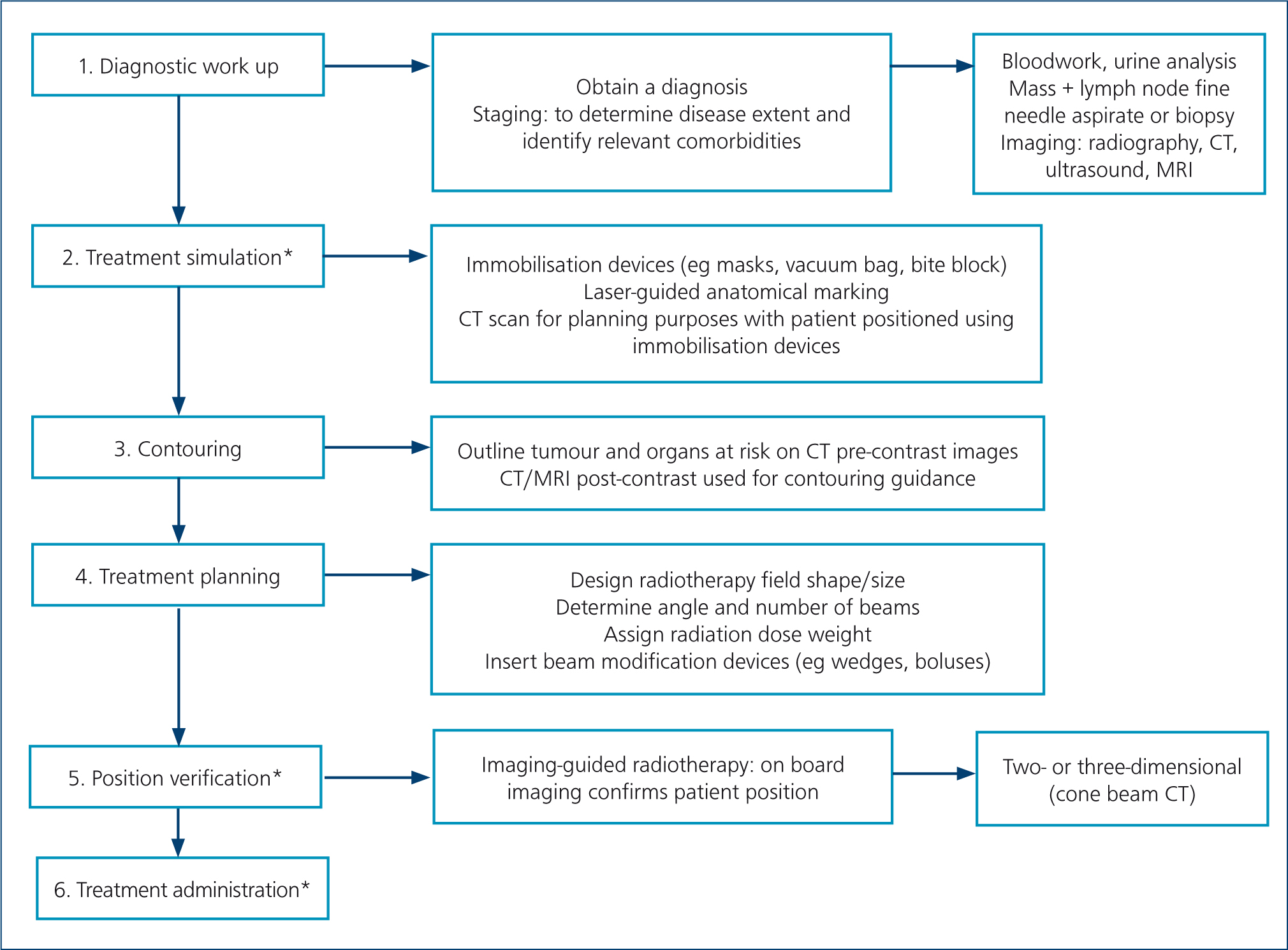

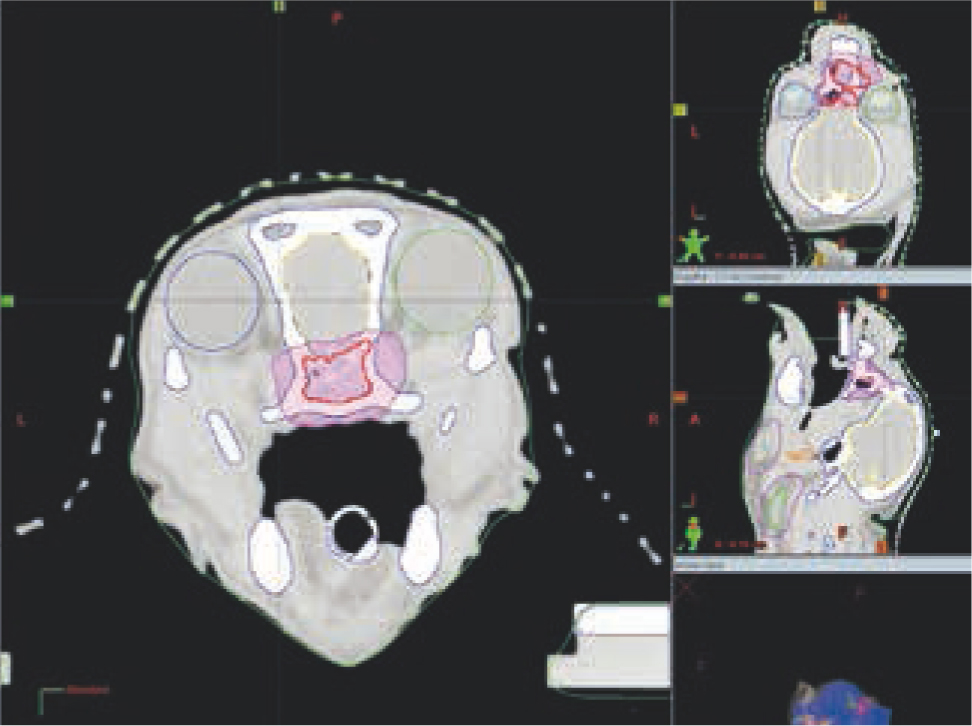



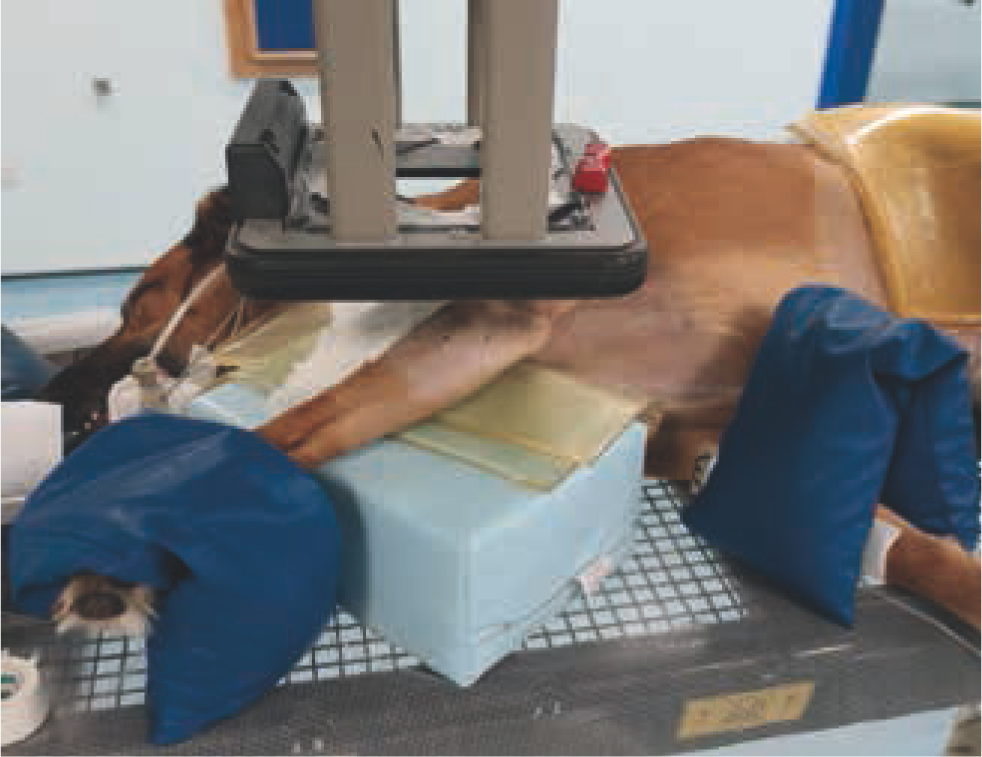
Radiation-induced toxicity in normal tissue
Normal tissue cells, similarly to cancer cells, are susceptible to the damaging effects of ionising radiation (Ward, 1988; Stewart and Dorr, 2009; Hill and Bristow, 2013). The risk, type, and severity of toxicity from radiotherapy depends on multiple factors including the tissue type, duration over which treatment is administered, volume of treated tissue, treatment fractionation, and the total dose delivered (Fowler, 1989; Schmidt-Ullrich et al, 1999; Purdy, 2008).
Adverse events induced by radiotherapy are broadly divided into acute or late toxicity effects (Emami et al, 1991; Gillette et al, 1995).
Acute adverse events
Acute adverse events are reversible, self-limiting effects typically seen during or shortly after radiotherapy. Tissues that rapidly proliferate (e.g. epithelium, mucous membranes, bone marrow) are most susceptible to acute toxicity effects (Hopewell, 1986; Harris et al, 1997). Table 2 outlines typical acute toxicities scored by the Veterinary Radiation Therapy Oncology Group (VRTOG) scheme (LaDue and Klein, 2001); Figures 8–10 give some examples.
Table 2. Veterinary Radiation Therapy Oncology Group acute radiation morbidity scoring scheme
Organ/tissue | 0 | 1 | 2 | 3 |
---|---|---|---|---|
Skin | No change over baseline | Erythema, dry desquamation, alopecia/epilation | Patchy moist desquamation without oedema | Confluent moist desquamation with oedema and/or ulceration, necrosis, haemorrhage |
Mucous membranes or oral cavity | No change over baseline | Injection without mucositis | Patchy mucositis with animal seemingly pain-free | Confluent fibrinous mucositis necessitating analgesia, ulceration, haemorrhage, necrosis |
Eye | No change over baseline | Mild conjunctivitis and/or scleral injection | Keratoconjunctivitis sicca requiring artificial tears, moderate conjunctivitis or iritis necessitating therapy | Severe keratitis with corneal ulceration and/or loss of vision; glaucoma |
Ear | No change over baseline | Mild external otitis with erythema, pruritus secondary to dry desquamation (not needing therapy) | Moderate external otitis (requiring topical medication) | Severe external otitis with discharge and moist desquamation |
Lower gastrointestinal tract | No change over baseline | Change in quality of bowel habits, rectal discomfort (not requiring medication) | Diarrhoea or rectal discomfort (requiring analgesia) | Diarrhoea requiring parenteral support, bloody discharge necessitating medical attention, fistula, perforation |
Urogenital | No change over baseline | Change in frequency of urination (not requiring medication) | Change in frequency urination (requiring medication) | Gross haematuria or bladder obstruction |
Lung | No change over baseline | Alveolar infiltrate cough: no treatment required | Dense alveolar infiltrate, cough: treatment required | Dyspnoea |
Central nervous system | No change over baseline | Minor neurological findings managed with prednisone therapy only | Neurological findings requiring intervention beyond prednisone therapy | Serious neurological impairment such as paralysis, coma, obtunded |
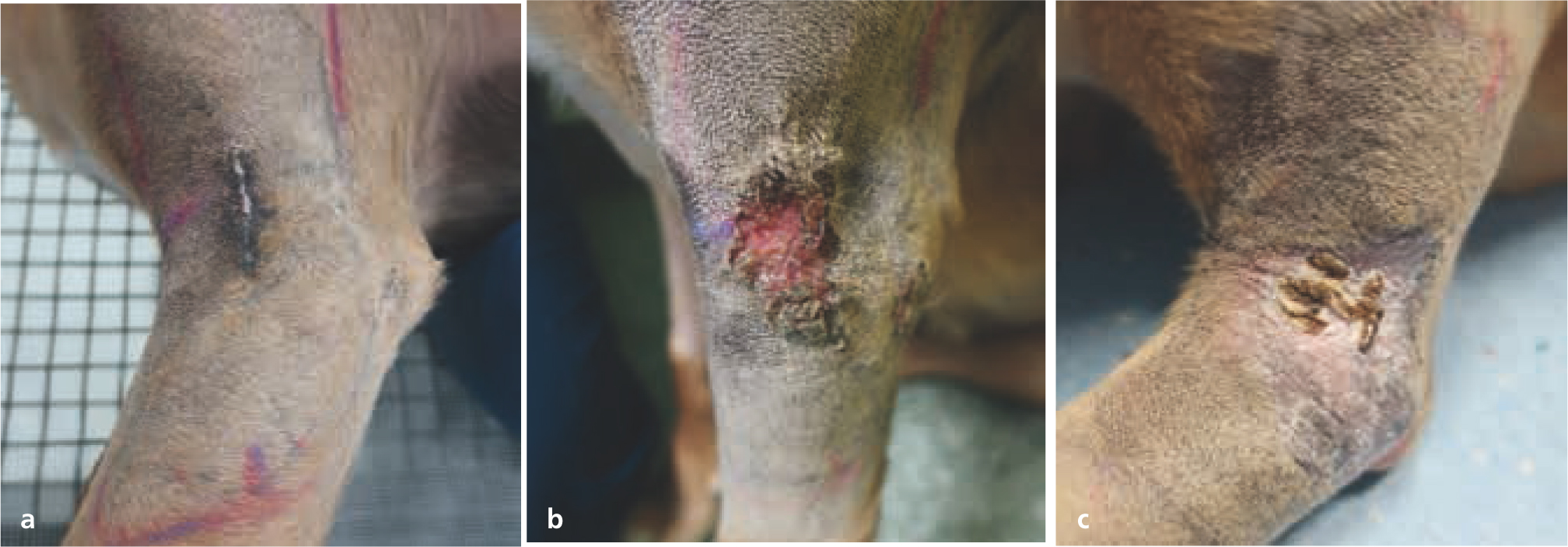
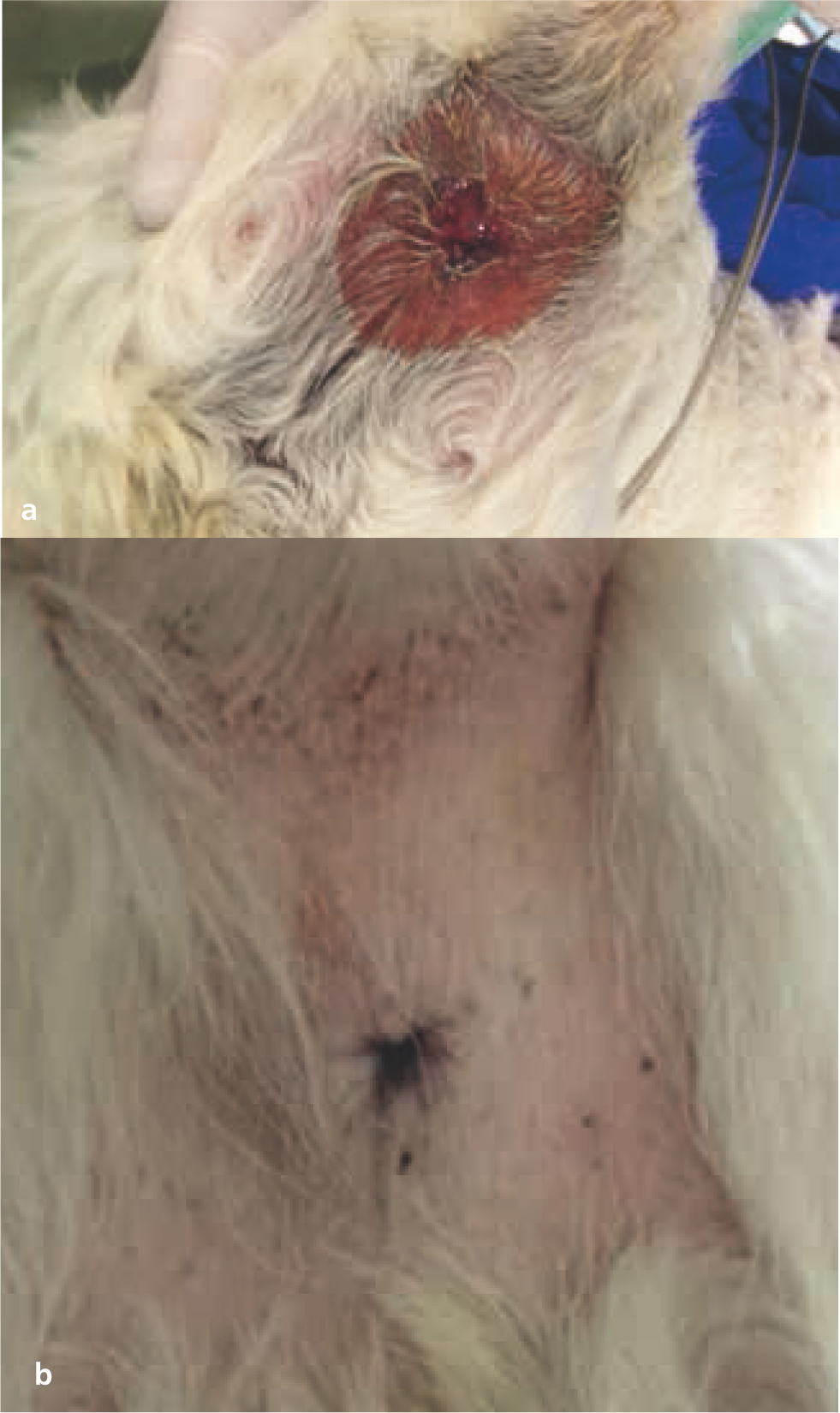
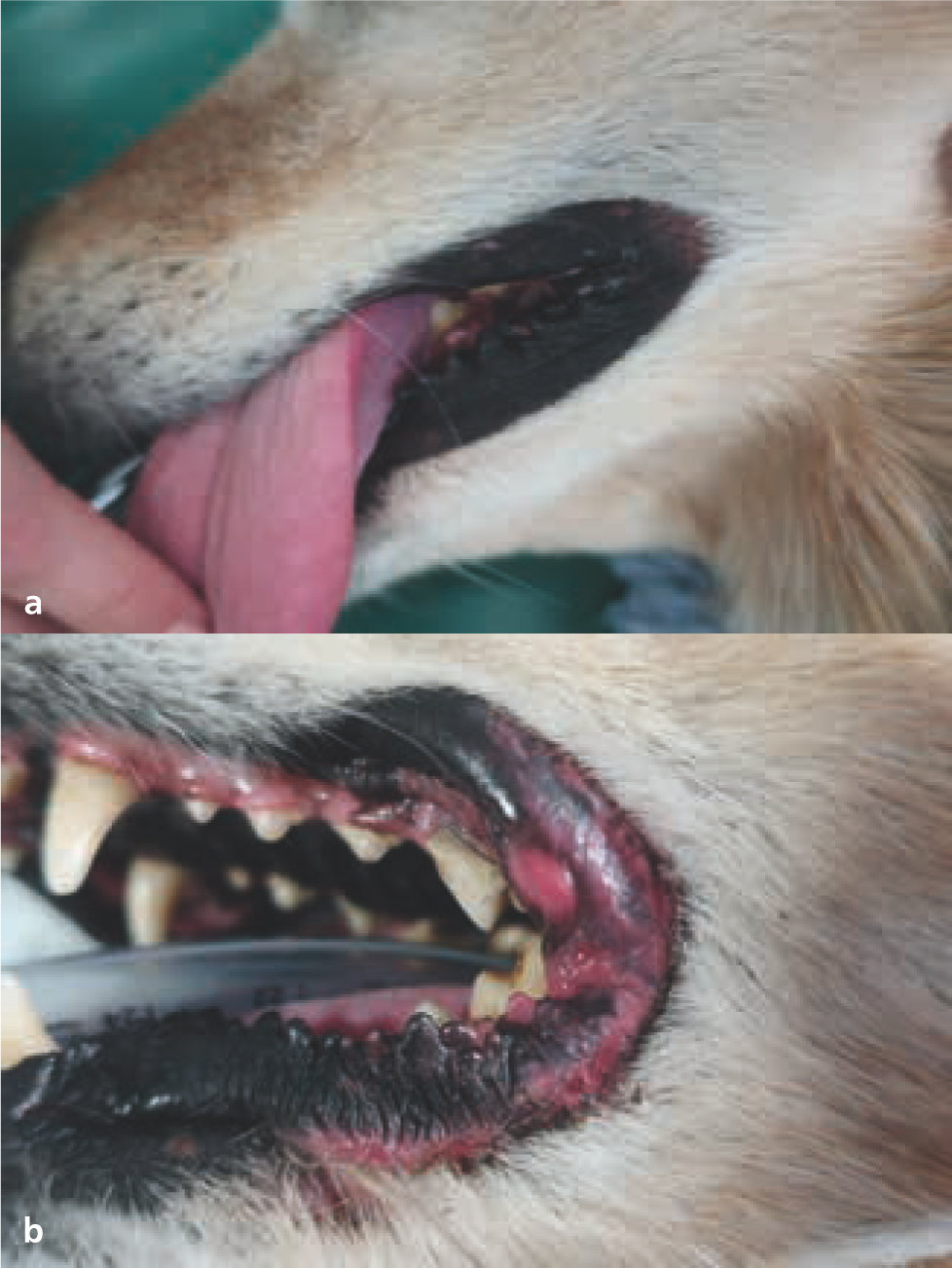
The severity of acute radiotherapy toxicity increases with higher total dosage and greater protocol intensity (higher doses given in a relatively shorter period). Increased tissue volume (e.g. skin) within the radiotherapy field can cause more debilitating adverse effects, prolonged healing time, and greater potential for infection. Bone marrow suppression is a rare complication in veterinary medicine, as radiotherapy is typically used as a local treatment; clinically relevant bone marrow toxicity only occurs when large areas of the body are irradiated, as other bone marrow sites can otherwise compensate (Hall and Giaccia, 2018).
Acute toxicity is self-limiting, and typically has fully resolved by 2–4 weeks after treatment (McEntee, 2006). Supportive care is essential to manage discomfort until the toxicity resolves. Currently, there are no established guidelines in veterinary medicine for management of acute toxicity caused by radiotherapy, but anti-inflammatory drugs and analgesics are most often used (Flynn and Lurie, 2007; Kumar et al, 2010; Ettinger et al, 2016). The authors prefer to use non-steroidal anti-inflammatory drugs to manage acute radiation-induced dermatitis, as these are better tolerated and have a stronger analgesic effect than steroids. Additionally, a prospective study found no reduction in severity of acute radiation-induced dermatitis when using steroids (Flynn et al, 2007).
Prophylactic antibiotic use remains controversial (Flynn and Lurie, 2007) and the authors typically treat only where a bacterial infection has been confirmed within the radiation site, based on cytology and bacterial culture. A study evaluating the effect of prophylactic cephalexin in dogs with radiation-induced dermatitis, reported that dogs receiving cephalexin experienced toxicities of greater severity and duration than the control group (Keyerleber and Ferrer, 2018). Although there was no difference in prevalence of bacterial infection compared to dogs not receiving antibiotics, dogs prophylactically treated had a higher prevalence of multidrug resistant infections.
Protection of the radiation field from additional external damage is another crucial part of toxicity management. The authors recommend avoiding self-trauma with Buster collars, minimising ultraviolet exposure in dogs or cats with radiation-induced dermatitis, and avoiding hard foods or toys in those receiving oral cavity radiation; oral rinses have been beneficial for managing oral mucositis in the authors' experience. The radiotherapy site should not be bandaged or rubbed. Table 3 outlines typical supportive medications used by the authors to palliate acute toxicity.
Table 3. Supportive treatment for the management of complications related to radiotherapy most commonly used at the authors' institution
Medication | Indication |
---|---|
Systemic administration (typically orally) | |
Steroidal anti-inflammatories | If brain or spinal cord is within the treatment field, to minimise risk of radiotherapy-related oedema |
Non-steroidal anti-inflammatory drugs | To manage radiation-induced inflammation and discomfort. Used as long as no contraindications for non-steroidal anti-inflammatory drug use and if steroids are not indicated |
Analgesics | Multimodal analgesia prescribed in an escalatory manner as required. Examples include gabapentin, paracetamol, buprenorphine, amantadine, codeine, tramadol and fentanyl patches in certain cases. Local nerve blocks can also be considered if required |
Topical administration | |
Topical oral rinses | Mouthwash containing antihistamine and local anaesthetic to relieve oral mucositis-related discomfort |
Topical eye lubricant | If eyes are within the treatment field, to decrease risk of keratitis or corneal ulcers |
Topical antiseptic | Protective creams such as silver sulfadiazine for radiation-induced dermatitis |
Late toxicities
Unlike acute toxicity, late normal tissue toxicities occur months to years after radiotherapy. They result from irreversible and progressive tissue fibrosis. Late toxicity affects slowly proliferating tissues (e.g. brain, muscle, spinal cord, nerve, bone, kidney, heart, and lung) because of vascular and stromal damage, chronic inflammation, fibrosis, necrosis, and loss of normal stem cells (Harris et al, 1997; Fajardo et al, 2001).
While most late radiotherapy toxicities are clinically inconsequential (e.g. hyperpigmentation or leukotrichia, Figure 11), each prescription and radiation plan is carefully designed to minimise clinically relevant and life-threatening late toxicities (e.g. radionecrosis, radiation-induced tumour development, or organ fibrosis) (Johnstone et al, 1995; Hosoya et al, 2008). Table 4 outlines typical late toxicities scored by the VRTOG scheme (LaDue and Klein, 2001). Radiation-induced neoplasia (e.g. sarcoma) is another rare (i.e. affecting less than 1% of treated animals) but potential late toxicity (Gillette et al, 1990; Hall and Wuu, 2003; Hosoya et al, 2008). The radiation oncologist's prescription doses and treatment plans are designed to give less than 5% risk of developing clinically relevant late toxicities (Ettinger et al, 2016; Withrow et al, 2019).
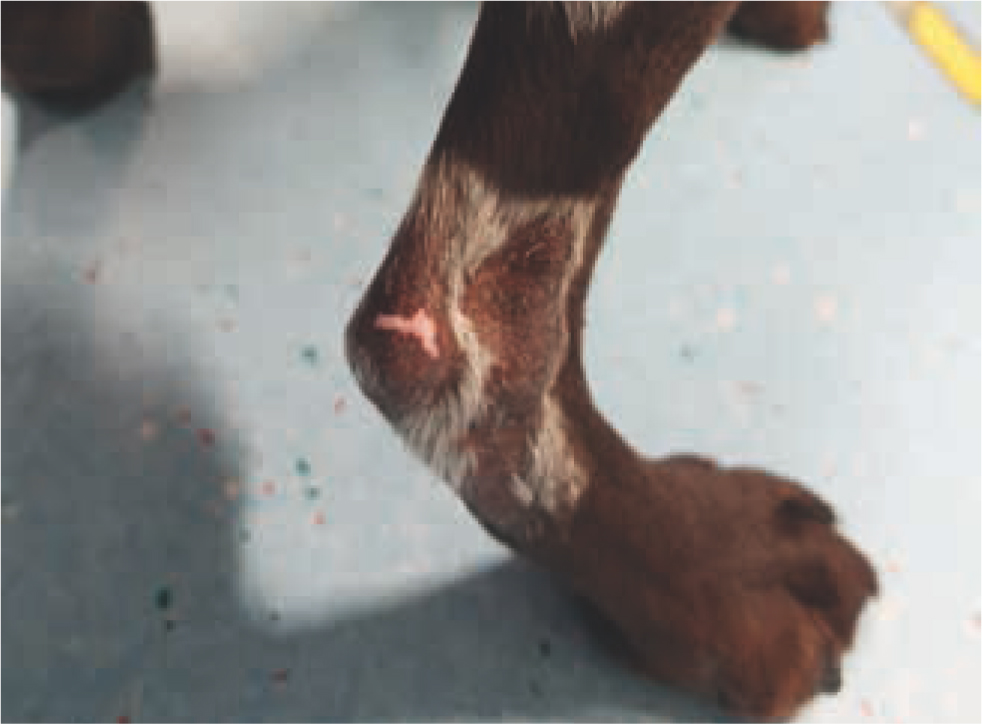
Table 4. Veterinary Radiation Therapy Oncology Group late radiation morbidity scoring scheme
Organ/tissue | 0 | 1 | 2 | 3 |
---|---|---|---|---|
Skin/hair | None | Alopecia, hyperpigmentation, leukotrichia | Asymptomatic induration (fibrosis) | Severe induration causing physical impairment, necrosis |
CNS | None | Minor neurological findings managed with prednisone therapy only | Neurological findings requiring intervention beyond prednisone therapy | Seizures, paralysis, coma |
Eye | None | Asymptomatic, cataracts, keratoconjunctivitis sicca | Symptomatic cataracts, keratitis, corneal ulceration, minor retinopathy, mild to moderate glaucoma | Panophthalmitis, blindness, severe glaucoma, retinal detachment |
Bone | None | Pain on palpation | Radiographic changes | Necrosis |
Lung | None | Patchy radiographic infiltrates | Dense radiographic infiltrates | Symptomatic fibrosis, pneumonitis |
Heart | None | Electrocardiogram changes | Pericardial effusion | Pericardial tamponade, congestive heart failure |
Joint | None | Stiffness | Decreased range of motion | Complete fixation |
Bladder | None | Microscopic haematuria | Pollakiuria, dysuria, haematuria | Contracted bladder |
The risk of late toxicity is predominantly dependent on fraction size, although total dose, organ radiosensitivity, and normal tissue volume irradiated also play a role in development (Withers et al, 1988). By fractionating (splitting) the total dose into a greater number of treatments, lower doses are given per fraction, enabling patients to tolerate higher total doses (Kaanders et al, 1992) without increasing the risk of clinically relevant late toxicities. Fractionation enables normal cells to repair sub-lethal DNA damage between fractions. In contrast, large dose administration in one treatment causes irreversible and lethal DNA damage with no option for repair (Nickoloff et al, 2017). Most tissues require a minimum inter-fraction interval of 6 hours for repair, although some tissues such as spinal cord require a longer time (24 hours) (Frindel et al, 1972; Hopewell et al, 1987; Joiner and Kogel, 2019). The volume of tissue as well as tissue type irradiated can significantly impact the risk of developing clinically relevant toxicities (Parmentier et al, 1983). For example, some organs with redundant functional units, such as the lungs and kidneys, may tolerate high doses to some of their total volume, as long as enough residual tissue remains functional. In contrast, serial organs such as the spinal cord, in which there is dependence on each unit, may lose function even if only a small segment is damaged (Emami et al, 1991; Pan et al, 2010; Boittin et al, 2015; Giridhar et al, 2015).
Applications of radiotherapy in veterinary oncology
Many different radiotherapy protocols have been described for treatment of canine and feline cancers. Protocols vary in number of fractions, dose per fraction, and total dose, therefore differing in likelihood of tumour control and risk of developing acute or late toxicities. As in human medicine, veterinary radiotherapy protocols are broadly divided into either ‘definitive intent’ or ‘palliative intent’ protocols, although classifications for veterinary patients are less clear than in human medicine (McEntee, 2006).
Definitive intent protocols
Definitive intent protocols aim to provide tumour control for as long as possible by administering a high total dose. As the goal of these protocols is long-term survival, it is vital to minimise risk of developing clinically-relevant late toxicities. Therefore, the high total dose needs to be fractionated into many small dose administrations. Definitive radiotherapy protocols are typically administered on a daily basis, Monday through Friday, although some institutions may treat on a Monday–Wednesday–Friday basis because of logistical limitations (McEntee, 2002). Twice-daily fractions can be administered in specific circumstances (e.g. where the animal has missed a treatment), as long as there is an adequate interval between fractions, to enable normal tissue repair (Angus and Piotrowska, 2014). The number of fractions varies by protocol but typically consists of 10–20 treatments given over 2–4 weeks.
Radiation induces logarithmic cell kill, making the probability of tumour control highly dependent on the number of tumour cells present at the start of the treatment. As such, radiotherapy is considered most effective for microscopic disease (Halperin et al, 2013). The radiotherapy can be administered either before (neoadjuvant) or following surgery (adjuvant). Table 5 compares the benefits of these approaches. A patient- and tumour-specific therapeutic plan, including the order of treatment modalities, should be carefully designed at the time of diagnosis, and involve multiple disciplines (medical oncology, radiation oncology and surgery) to optimise decision making.
Table 5. Advantages and disadvantages of preoperative vs postoperative radiotherapy
Advantages | Disadvantages | |
---|---|---|
Preoperative radiation therapy |
|
|
Postoperative radiation therapy |
|
|
Neoadjuvant radiotherapy
Neoadjuvant radiotherapy can be considered in large infiltrative tumours in which complete excision is not feasible (Brearley et al, 1999; Brearley, 2000; Eckstein et al, 2009). Neoadjuvant radiotherapy is most commonly used for feline injection site sarcomas (Kobayashi et al, 2002) (Figure 12). Owing to the highly infiltrative nature of feline injection site sarcomas, wide surgical margins (i.e. 5 cm lateral margins and two fascial planes deep) are recommended in order to achieve adequate local control (Phelps et al, 2011). These aggressive margins are often extremely challenging to achieve unless the tumour is small. Preoperative radiotherapy can enable more conservative surgeries by ‘sterilising’ the tumour cells infiltrating the normal tissue around the mass (Kobayashi et al, 2002). Occasionally, radiotherapy can also shrink the mass, further facilitating surgery (Nolan et al, 2013).

Adjuvant radiotherapy
Adjuvant radiotherapy is frequently used to treat the microscopic disease that has been left behind following incomplete excision of several tumour types, and it significantly increases long-term local tumour control in canine mast cell tumours and soft tissue sarcomas (Figure 13) (McChesney et al, 1989; Frimberger et al, 1997; LaDue et al, 1998; Forrest et al, 2000; Chaffin and Thrall, 2002; Dobson et al, 2004; Hahn et al, 2004; Kry and Boston, 2014). Placement of surgical clips to delineate the tumour bed margins can make radiation planning much less challenging (McEntee et al, 2008). Adjuvant radiotherapy should be started as soon as possible after surgery; however, the surgical site must be healed before radiotherapy starts, to avoid wound dehiscence. Surgical flaps should be avoided if the plan is to irradiate the surgical site, as they are at increased risk of dehiscence even if fully healed before radiotherapy, and can significantly increase the size of the radiotherapy field (Séguin et al, 2005).
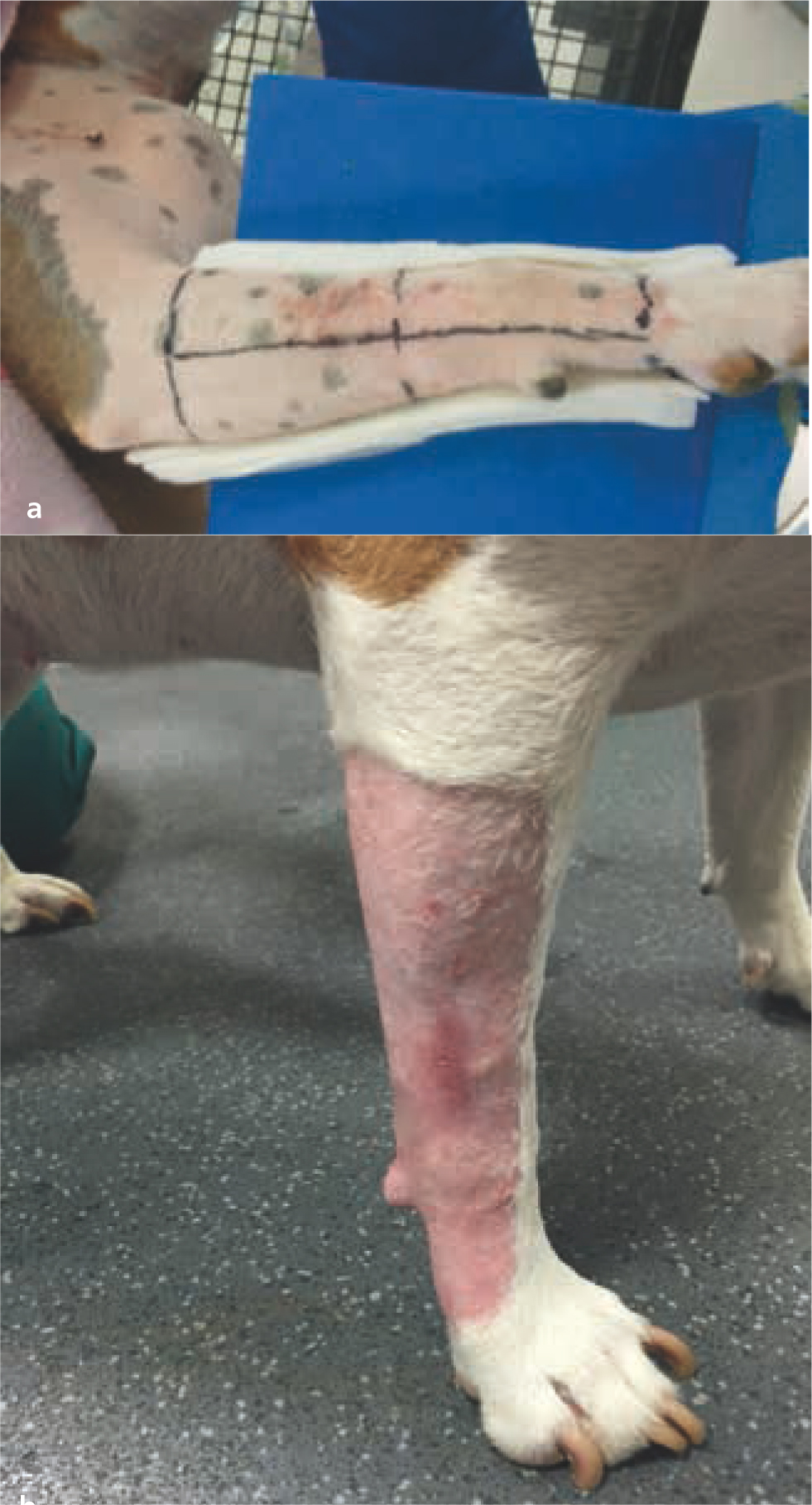
Definitive radiotherapy is also considered the sole treatment for some tumour types, particularly where surgical intervention is ineffective or limited (Withrow et al, 2019). Radiotherapy is considered ‘gold standard’ treatment for nasal tumours in veterinary patients, as it provides better local control than surgery (Henry et al, 1998). Clinical resolution or marked improvement of clinical signs is expected in the majority of the treated animals. Additionally, radiotherapy can stabilise or reduce tumour size, although this is temporary in most cases (Morgan et al, 2018); (Figure 14). Definitive radiotherapy is also used as a primary modality in veterinary patients with intracranial tumours (Hu et al, 2015). With the exception of specific tumour types and locations (e.g. surgical excision is considered gold standard for feline meningiomas; Gordon et al, 1994), surgery has not been shown to be more effective than radiotherapy as a primary treatment in veterinary patients (Hu et al, 2015). Surgical excision of intracranial masses is not always feasible and can be controversial in instances where surgery is possible, because of the associated risk of morbidity (Motta et al, 2012; Körner et al, 2019b). Radiotherapy has been shown to effectively manage both extra-axial and intra-axial tumours in dogs and cats (Figures 15 and 16). Most animals enjoy marked clinical improvement after radiotherapy and do not develop clinically relevant radiotherapy toxicities as long as appropriate dose planning and dose prescription are performed (Hu et al, 2015; Schwarz et al, 2018). As such, the authors consider radiotherapy to be the preferred modality for managing most intracranial tumours in pets.
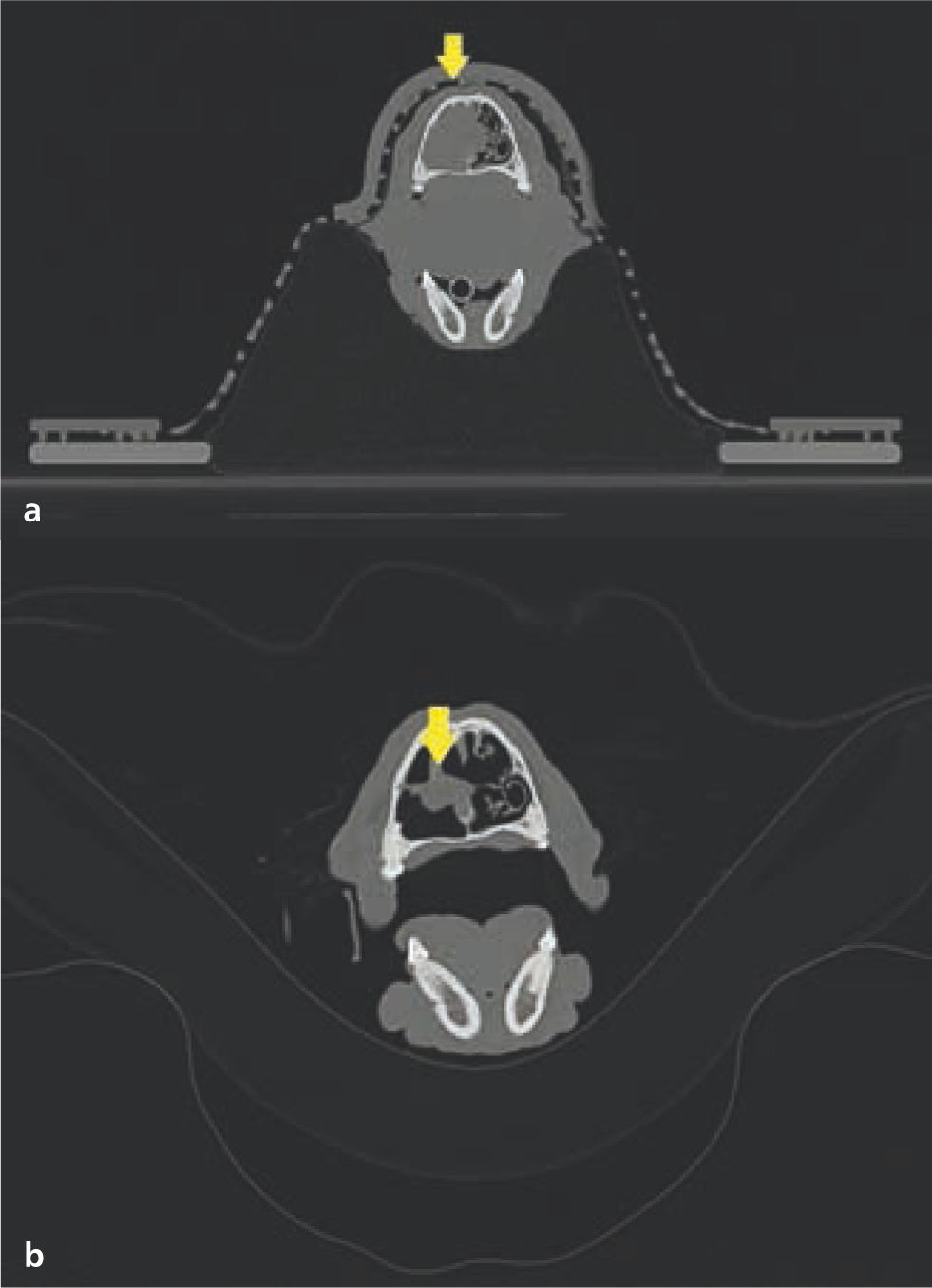
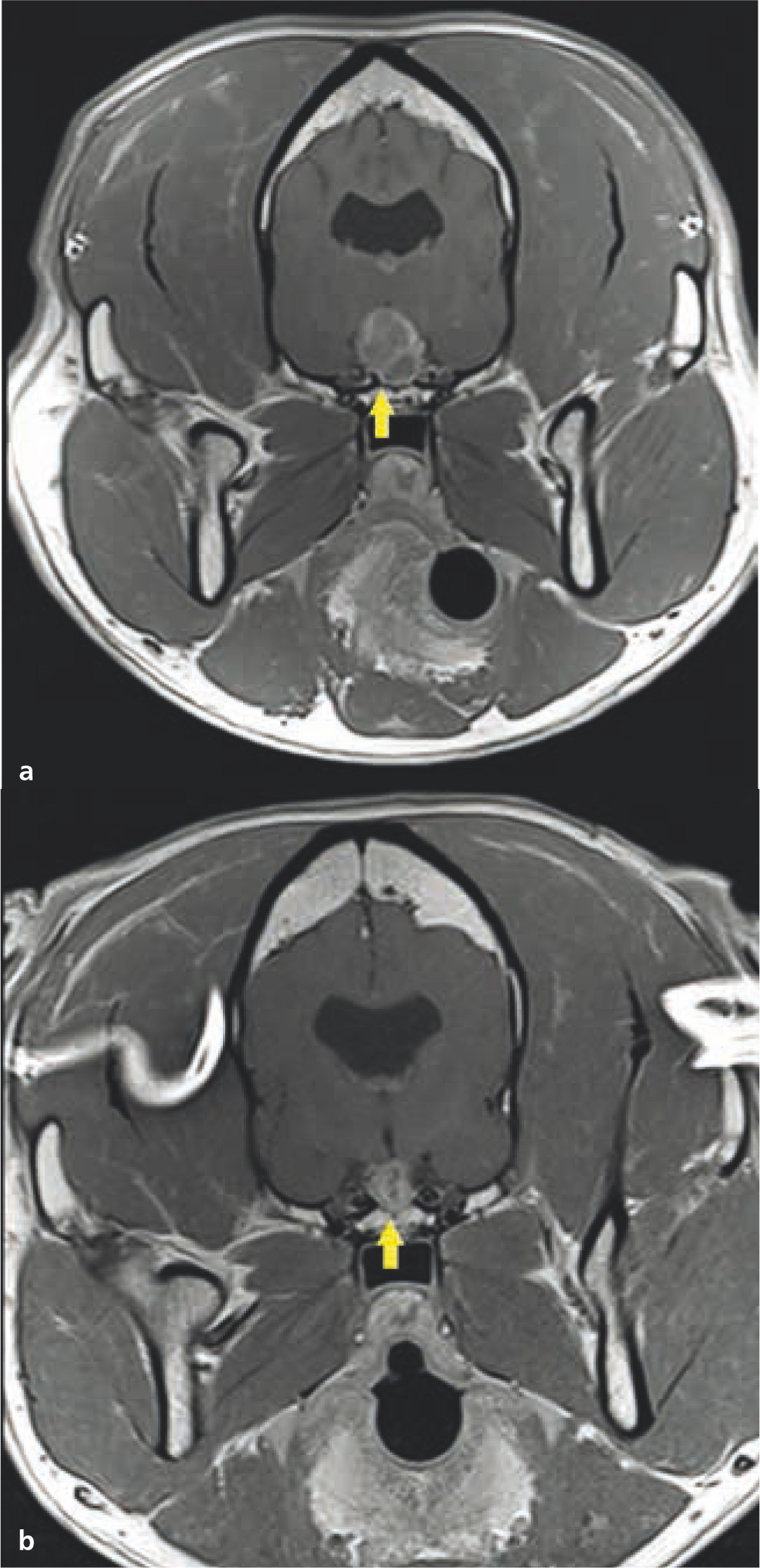
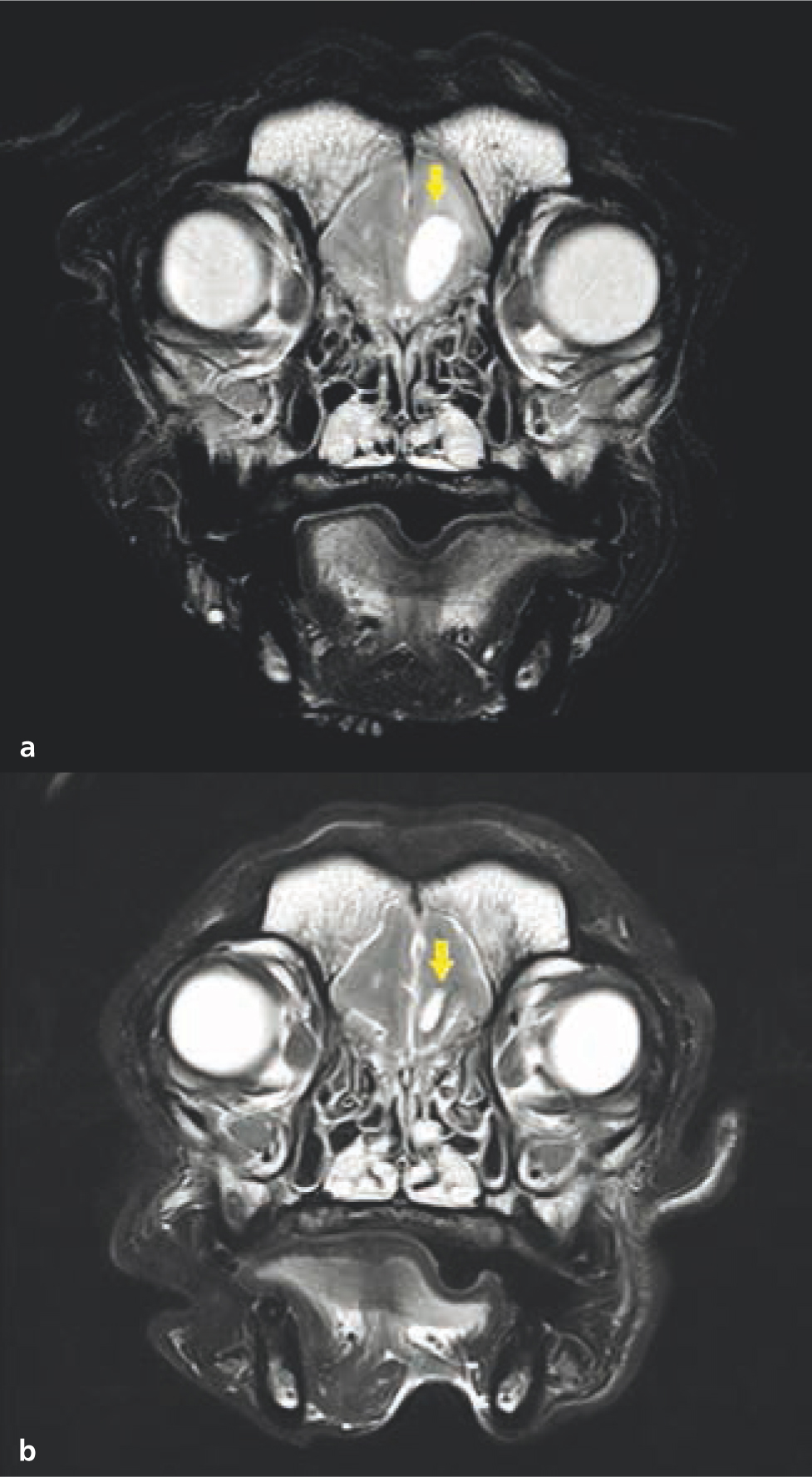
Palliative intent protocols
The aim of palliative radiotherapy protocols is to improve the quality of life of patients with advanced stage disease, by reducing pain or associated inflammation, and by resolving or improving clinical signs secondary to their neoplasia. Tumour control can occur, and often occurs to some extent with palliative protocols (Figures 17 and 18); however, this is not the primary goal of treatment (Thrall and LaRue, 1995; Tollett et al, 2016).
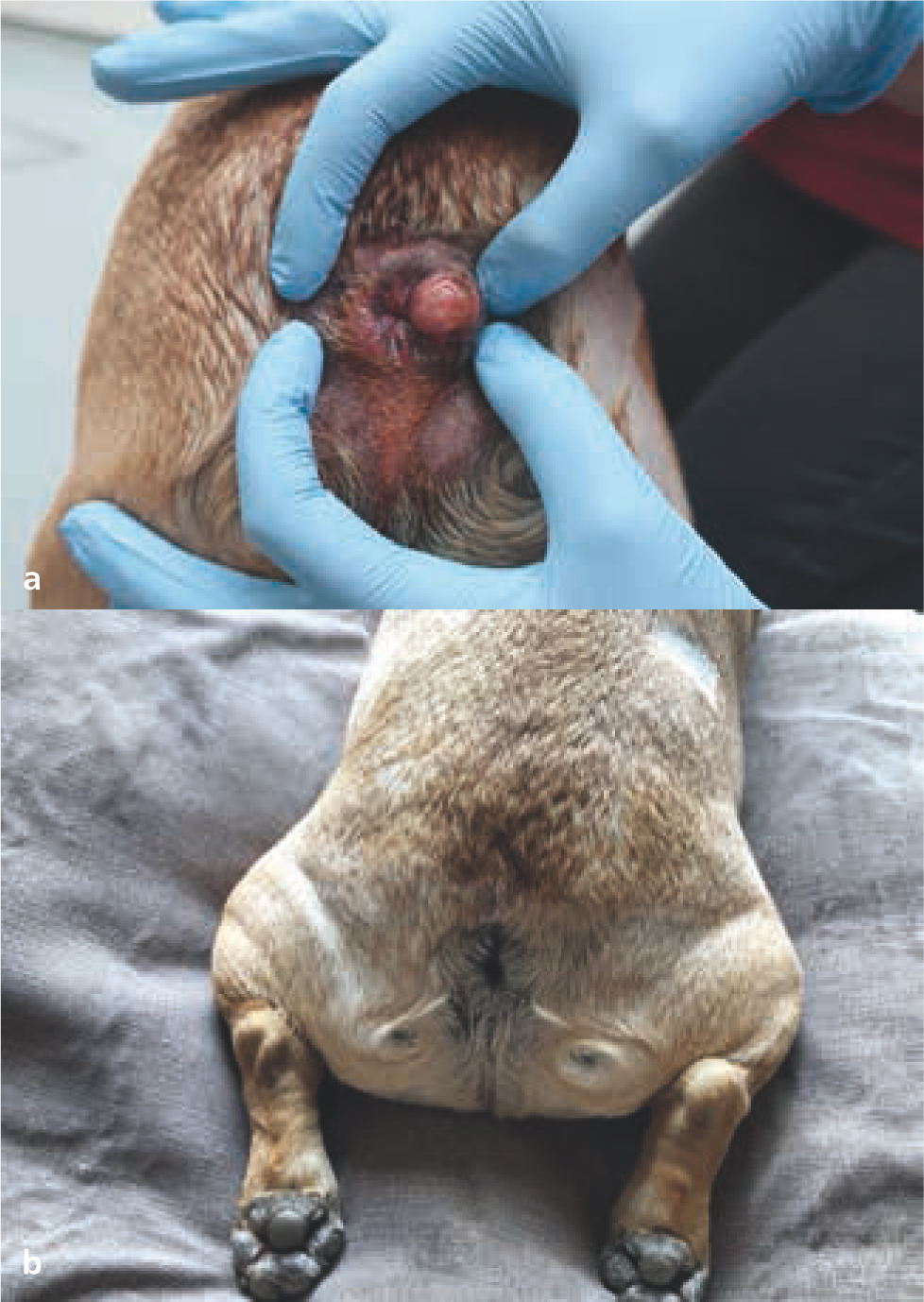
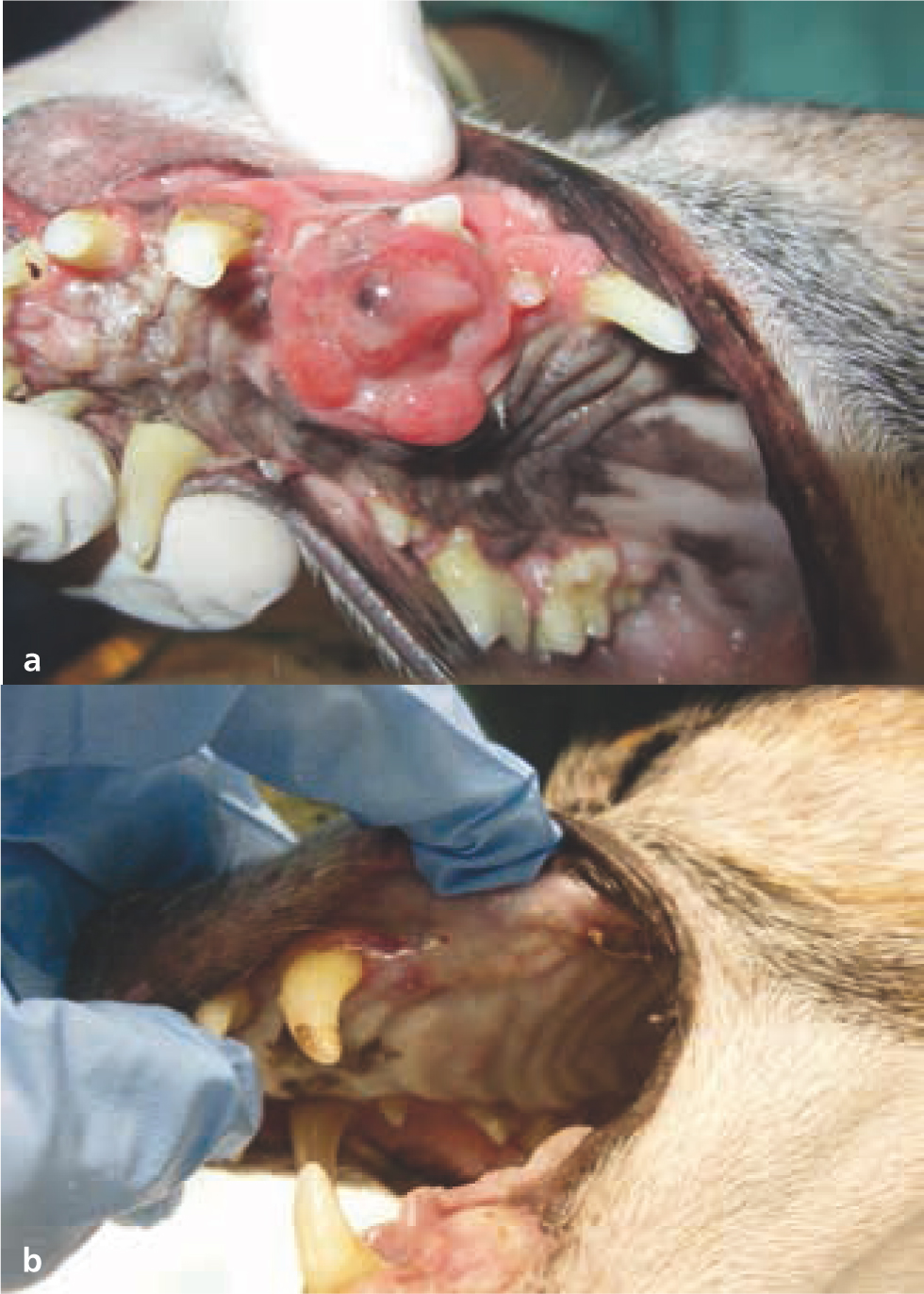
Palliative protocols are typically hypofractionated (i.e. higher doses per fraction given over fewer treatment fractions compared to definitive protocols) with two to five fractions given either daily or weekly. Hypofractionated protocols are generally less expensive and demanding for both owners and pets, because of the reduced number of treatments, general anaesthetics, and hospital visits (McEntee, 2004). Except for some specific exceptions (such as melanoma) (Freeman et al, 2003), hypofractionated palliative protocols are considered less effective than definitive intent protocols in terms of tumour control (Nishiya et al, 2016).
Palliative protocols deliver a lower total dose in order to prevent or minimise risk of developing acute toxicities. The severe acute toxicities associated with definitive intent protocols (e.g. mucositis, dermatitis) can cause discomfort for weeks and are not appropriate in a palliative setting for animals with a guarded to poor prognosis in the short term. In contrast, palliative protocols can increase the risk of developing life-threatening late toxicities that occur months to years after radiotherapy (McEntee, 2006; LaRue and Custis, 2014). Repeating a palliative protocol can be considered in animals enjoying good durable responses (e.g. improved quality of life for 6 or more months); however, the second response duration is typically shorter and the risk of late toxicity is further increased.
In particular, palliative radiotherapy is frequently used for management of tumour-related bone pain; although the exact mechanisms for its effectiveness are unknown, proposed mechanisms include decreased osteoclast activity, alteration of inflammatory chemical mediators, and decreased mass size reducing pressure on nearby endosteum or nerves (Vakaet and Boterberg, 2004; De Felice et al, 2017). Radiotherapy can provide improved pain control and limb function in dogs with appendicular osteosarcoma where surgery is declined or not possible (McEntee et al, 1993; Ramirez et al, 1999). The presence of pulmonary metastases is not a contraindication to palliative radiotherapy, as long as the dog is not showing clinical signs related to their metastatic disease. Median time to improvement in limb function after radiation has been reported as approximately 2 weeks, and typically lasts 1–5 months (Dernell et al, 2000; Knapp-Hoch et al, 2009). The authors consider repeating palliative radiotherapy in patients who experienced 6 months or more of adequately controlled pain; however, the risk of pathological fracture inherent to osteosarcoma increases with the total dose and time from initial treatment administration (Figure 19).
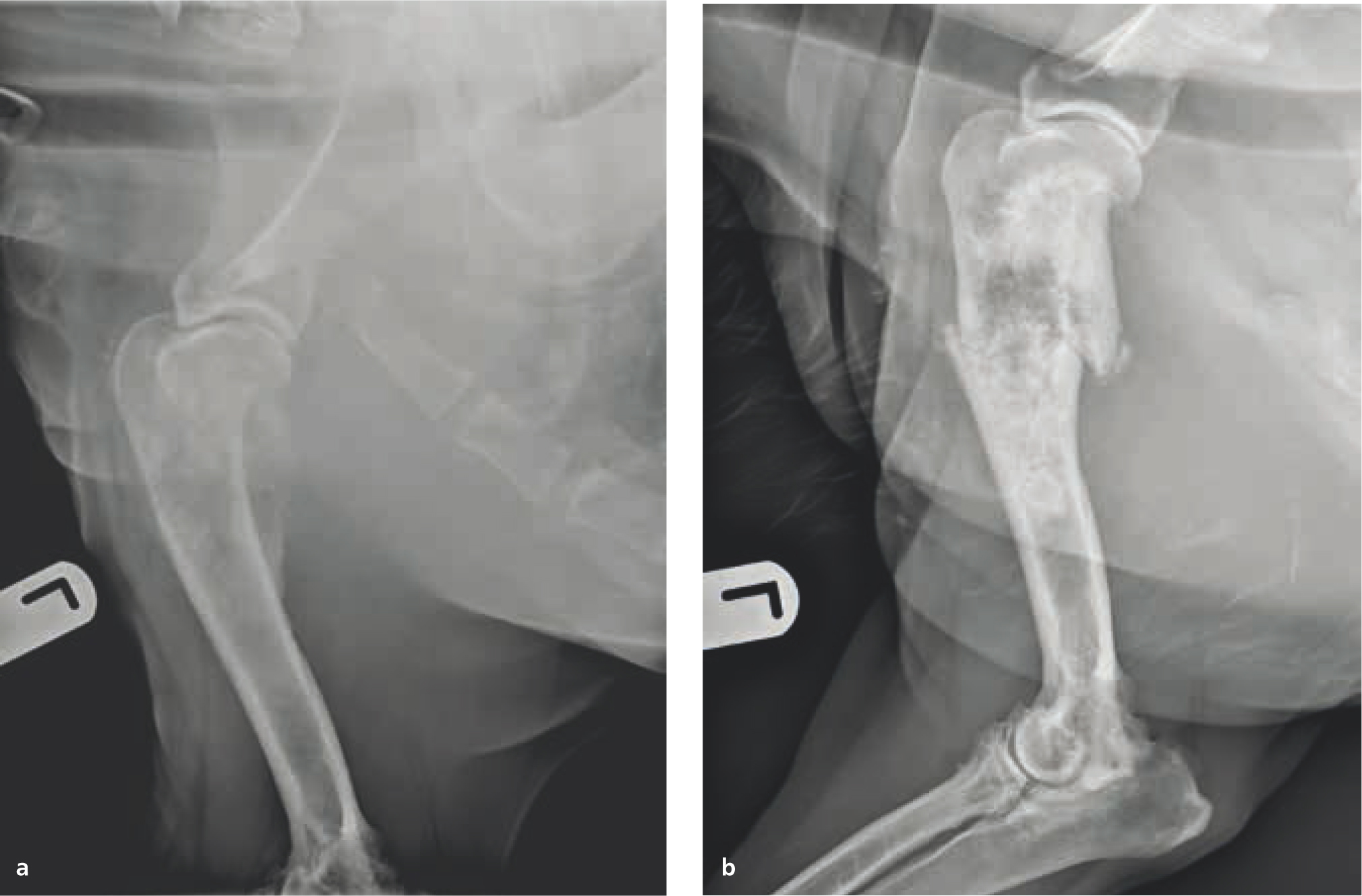
Palliative radiotherapy is also frequently considered in advanced stage nasal tumours (i.e. with cribiform plate infiltration), as some studies have suggested that outcomes for palliative protocols are similar to those seen with the more demanding definitive intent protocols (Adams et al, 2009; Buchholz et al, 2009). Improvement or resolution of clinical signs such as poor air flow, epistaxis, nasal discharge, pain, exopthalmosis, and neurological signs are commonly seen after palliative radiotherapy of these tumours.
Ionising radiation in management of non-neoplastic conditions
Although radiotherapy is primarily used for tumour treatment, ionising radiation plays an important but niche role in managing specific non-malignant conditions (Seegenschmiedt, 2000; Micke et al, 2002). The underlying pathways are not fully understood (Montero Luis, 2013); however, potential biological mechanisms include radiation-induced changes in capillary permeability, destruction of inflammatory cells, modification of cytokine expression, and anti-proliferative effects (Hildebrandt et al, 1988, 2000; Trott, 1994; Trott et al, 1995; Trott and Kamprad, 1999; Kern et al, 2000).
Clinical oncologists were initially reticent to consider ionising radiation as a therapeutic modality for benign conditions in humans, because of the inherent risk of radiation-induced carcinogenesis (Order and Donaldson, 1998). Strict criteria should be considered to identify human and veterinary patients where treatment benefits outweigh the potential (Montero Luis, 2013):
- The benign disorder must impact the patient's quality of life significantly in order to justify the risks of developing radiotherapy toxicities
- The benign disorder must be refractory to conventional therapy and ionising radiation is considered the safest or most effective next therapeutic alternative
- The minimum effective field size, dose per fraction, and total dose should be used to minimise risks
Ionising radiation has been shown to effectively manage multiple benign canine disorders that were refractory to conventional treatments, including degenerative osteoarthritis (Kapatkin et al, 2016; Rossi et al, 2018), meningoencephalitis of unknown origin (Beckmann et al, 2015; Körner et al, 2019a) and salivary mucocoeles (Poirier et al, 2018). Preliminary results have been promising and warrant further evaluation of radiotherapy in the management of these conditions (Table 6). An earlier study also described the benefits of ionising radiation for management of canine lick granulomas (Owen, 1989). Reports evaluating the use of radiotherapy for benign feline conditions are currently lacking. Nevertheless, cats suffering from painful inflammatory conditions such as lymphoplasmocytic rhinitis, gingivostomatitis or osteochondrodysplasia should be considered as radiotherapy candidates if non-responsive to conventional treatments (Hubler et al, 2004; Fujiwara-Igarashi et al, 2015).
Table 6. Published results of the use of radiotherapy for benign conditions
Medical condition | Protocol | Toxicity | Response | Reference |
---|---|---|---|---|
Lick granuloma | 2–4 fractions of 9–10 Gy, once weekly | Not reported | 92% complete response | Owen (1989) |
Sialocele | 3–5 fractions of 4 Gy each for a total dose of 12–20 Gy given in 1–3 fractions per week | No clinically relevant toxicities reported | 100% dogs benefited from treatment: 54% complete response, 45% partial response | Poirier et al (2018) |
Osteoarthritis | 3 fractions of 2 Gy over 3–5 days | No clinically relevant toxicities reported | Clinical improvement in 92% of dogs with median benefit duration of 1 year | Rossi et al (2018) |
Meningoencephalitis of unknown origin | 30 Gy delivered to whole brain over 2 weeks in 10 daily fractions of 3 Gy | No clinically relevant toxicities reported | 79% dogs benefited from treatment: 43% complete response, 36% partial response | Körner et al (2019a) |
Conclusions
Radiotherapy has become an integral therapeutic tool in veterinary medicine. It can provide long-term control over many tumours for which there was previously no effective treatment, and has shown excellent promise as a palliative treatment for patients with advanced stage neoplasia. Novel uses of radiotherapy include rescue therapy for specific benign conditions that are refractory to conventional therapy. Acute and late radiotherapy toxicities depend on the prescribed protocol as well as the organs within the radiation field; while typical protocols are designed to reduce these risks as much as possible, potential risks should be fully discussed with owners before commencing radiotherapy. Technical advances in the radiotherapy field may come at a higher cost; however, they have significantly improved safety, accuracy and efficacy of this treatment modality for veterinary patients.
KEY POINTS
- Radiotherapy is a fundamental treatment modality for cancer. There are multiple types of radiation treatments, which vary by administration route, therapy intent and type of ionising radiation or energy. Each type has a preferential indication, depending on the desired dose distribution.
- Acute adverse events are reversible, self-limiting and typically seen during or shortly after radiation treatment. They affect rapidly proliferating tissues, and increase with total dose and treatment intensity.
- Late adverse effects develop months to years after radiotherapy and affect non-proliferating or slowly proliferating tissues. It is vital to minimise the risk of clinically-relevant late toxicities, as these types of adverse effects are irreversible and can be life threatening.
- Conventional fractionated definitive protocols aim to provide long-term tumour control: total dose administered is high, but divided into many fractions in order to minimise risks of clinically relevant late toxicity.
- Palliative protocols aim to improve the quality of life for patients with cancer, by relieving pain and resolving or improving clinical signs caused by the tumour. These protocols typically deliver a lower total dose, with fewer fractions, but a higher dose per fraction than definitive intent protocols.
- Radiotherapy may provide an alternative treatment for benign conditions which are refractory to standard therapy, such as osteoarthritis, meningoencephalitis of unknown origin, and salivary mucoceles.