Products to controls slugs and snails are widely used by gardeners to reduce damage to plants but also by dog owners in efforts to reduce the risk of lungworm (Angiostrongylus vasorum). Slugs and snails carry lungworm larvae and dogs can become infected when they play with and consume the molluscs. Dogs are also at risk from exposure to lungworm larvae in the slime of slugs and snails through eating grass, drinking from puddles, outdoor water bowls or toys that have been left outside in grass.
The risks of exposure to the different compounds used to control slugs and snails are discussed in this article. It should be noted that there will be changes in the products available in the UK for slug and snail control (Figure 1), as there is a move away from the more toxic compounds commonly used in the past.
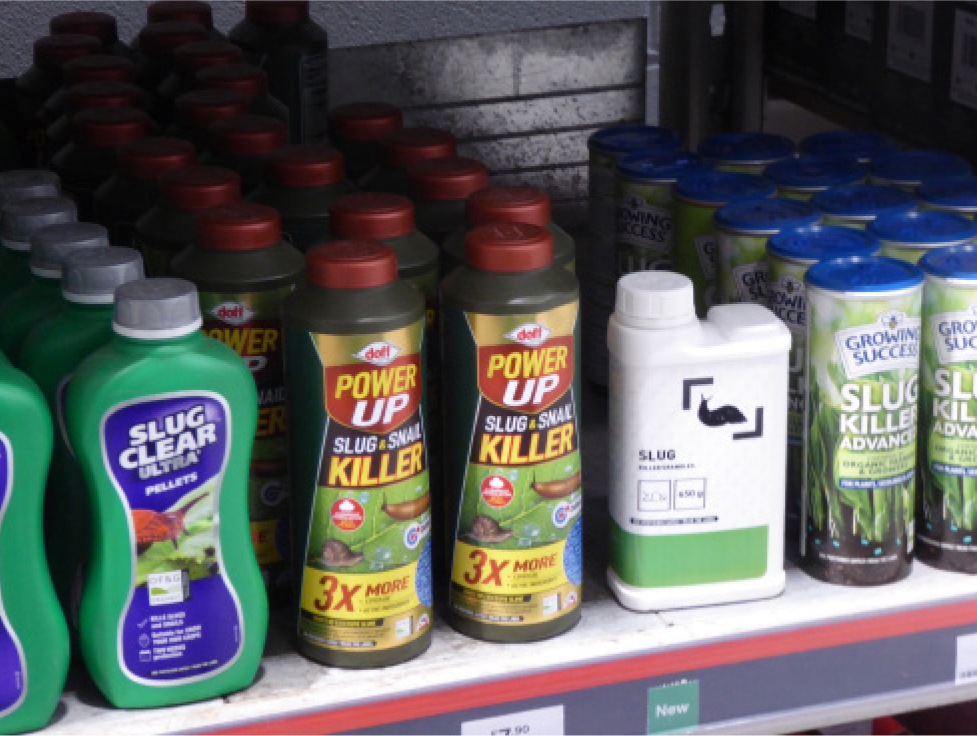
Slug baits are typically pellets which are usually a blue-green colour to deter birds (Turner, 2007). Studies have shown birds tend to avoid blue-coloured seeds (Pawlina and Proulx, 1996; Avery et al, 1999). The dye may also colour vomit and faeces.
Metaldehyde
Metaldehyde has long been a common ingredient of molluscicide preparations. However, from 31 March 2022 the outdoor use of metaldehyde slug baits has been banned in the UK to protect wildlife and the environment (Department for Environment, Food and Rural Affairs, 2020). This is also good news for pets, although it may be some time before cases of metaldehyde poisoning in pets become rare.
Metaldehyde-containing slug baits are usually small the blue-green coloured pellets, containing about 1.5–8% w/w metaldehyde in a bran/wheat filler. Most products contain 1.5% or 3% metaldehyde (Figure 2). Early research determined the lethal dose of metaldehyde in 50% of dogs (LD50) to be 210 mg/kg, with a range of 190–240 mg/kg (Poul and Rioche, 1960). Later sources quote the minimum lethal dose in dogs as 100 mg/kg and the LD50 as 100–1000 mg/kg (Booze and Oehme, 1985). Therefore, the individual dog's response may vary. In an experimental study, seven of eight dogs given 600mg/kg survived with no intervention but one died after 4.5 hours (Booze and Oehme, 1986). Unpublished data from the American Society for the Prevention of Cruelty to Animals (ASPCA) Animal Poison Control Center (APCC) suggest a treatment threshold for metaldehyde in dogs for doses of 2 mg/kg or more (Dolder, 2003)
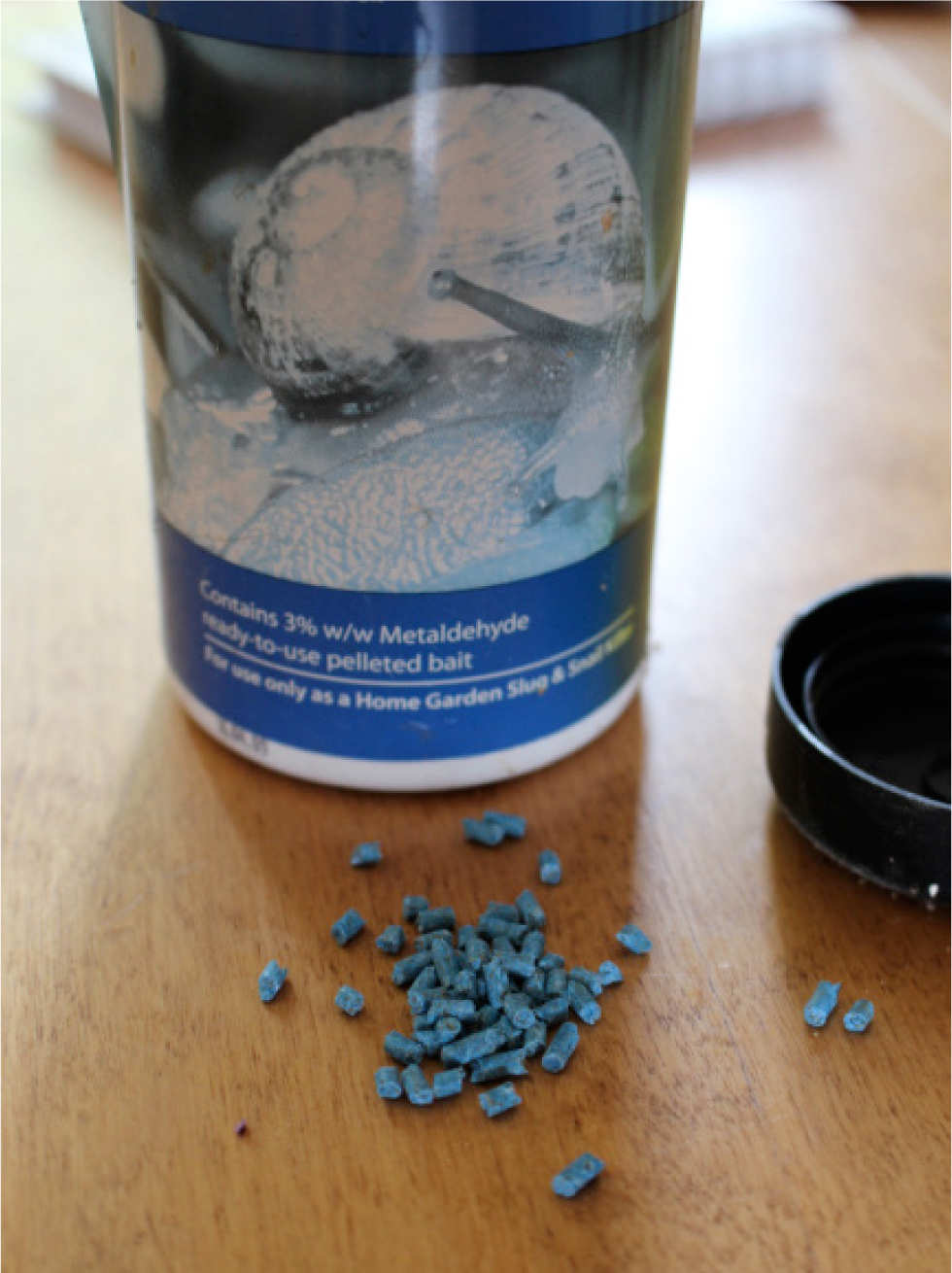
.
Mechanism of toxicity
Metaldehyde is thought to kill slugs and snails by causing dehydration and paralysis (Booze and Oehme, 1986). The mechanism of metaldehyde toxicity in mammals is not clearly understood. Metaldehyde is a tetramer of acetaldehyde and it was previously thought that metaldehyde undergoes hydrolysis by gastric acid to form acetaldehyde and that this is the primary toxic agent in metaldehyde poisoning. The clinical relevance of this process is doubtful. Although acetaldehyde was detected in the stomach contents of two dogs that died of suspected metaldehyde poisoning (Udall, 1973), it has not been detected in the serum, urine (Booze and Oehme, 1986; Shintani et al, 1999) or brain tissue (Sparks et al, 1996) of experimental animals after they ingested metaldehyde. In addition, the signs of poisoning after metaldehyde and acetaldehyde ingestion were different in experimental studies in mice (Sparks et al, 1996).
In mice, metaldehyde exposure has been linked to a decrease in the concentrations of the inhibitory neurotransmitter gamma-aminobutyric acid (GABA), noradrenaline and serotonin (5-hydroxytryptamine, 5-HT), as well as increased monoamine oxidase activity (Homeida and Cooke, 1982a, 1982b). Disruption of the GABAergic system may cause the convulsant activity seen with exposure to metaldehyde (Homeida and Cooke, 1982b; Sparks et al, 1996). It may be metaldehyde itself or an unknown metabolite that is responsible for the effects observed in metaldehyde poisoning.
Hyperthermia resulting from the increased muscular activity may also be a significant factor in metaldehyde toxicosis, as a pronounced rise in body temperature results in rapid cellular necrosis. Severe metabolic acidosis also occurs and may be the underlying cause of the hyperpnoea and central nervous system depression seen in cases of severe poisoning.
Clinical effects
Onset of clinical signs after ingestion of metaldehyde may be very rapid, often within 30 minutes of ingestion, but may be delayed for up to 3 hours. In a review of Veterinary Poisons Information Service (VPIS) cases, 50% of dogs developed signs within an hour of ingesting metaldehyde and recovery took on average 39 hours (range 1–120 hours) (Bates et al, 2012). Death can occur in a few hours but, on average in the VPIS cases, death occurred 18 hours after ingestion (range 1–36 hours) (Bates et al, 2012).
Common signs of metaldehyde toxicosis are hypersalivation, vomiting, diarrhoea (this can be discoloured blue-green), ataxia, panting, tremor, twitching, muscle spasms or fasciculation, seizures, opisthotonus and pyrexia. Respiratory effects (tachypnoea, dyspnoea, hyperventilation and respiratory depression) are less common and cardiac effects, including tachycardia or bradycardia, occur in roughly 10% of dogs (Bates et al, 2012).
Metaldehyde-induced seizures can last up to 72 hours but on average last 15 hours (Bates et al, 2012). Other potential toxicological causes of seizures in dogs can be seen in Box 1. Complications of prolonged seizure activity include severe pyrexia, rhabdomyolysis, disseminated intravascular coagulation and multi-organ failure.
Box 1.Potential toxocological causes of seizures in dogs
- Metaldehyde
- Tremorgenic mycotoxicosis
- Illicit drugs such as amphetamines
- Muscarinic mushrooms
- Pyrethrin and pyrethroid toxicosis
- Organophosphate insecticides
- Methiocarb and other carbamates
- Methylxanthines (eg caffeine, theobromine)
- Amitraz
- Strychnine
- Bromethalin (not approved in the UK or Europe)
- Chlorinated hydrocarbons
- Sodium monofluoroacetate/1080 (not available in the UK)
- Aluminium phosphide
Case report 1. Metaldehyde.
Within an hour of having access to a 15 kg bag of 3% metaldehyde on a farm, a 10-year-old cocker spaniel weighing 15 kg developed nystagmus, frothing at the mouth and pyrexia. Seizures started at 1.5 hours and blue stools were passed at 2 hours. The dog was given a gastric lavage and an enema and a lot of blue material was retrieved. Seizures were controlled with anaesthesia and then the dog was weaned off this. Midazolam was given when the dog became unsettled and when she had an isolated seizure. The dog then developed hypothermia and hyperaesthesia, and seemed disorientated, confused and blind. She was maintained on intravenous fluids and appeared well by 36 hours, although the blindness lasted 56 hours (VPIS case).
Metabolic acidosis is a common laboratory finding in metaldehyde toxicosis. Elevated liver enzymes can occur (probably caused by stress or barbiturate therapy). Elevated creatine kinase and lactate dehydrogenase can occur as a result of increased muscle activity, while renal impairment, although not common, is usually secondary to pyrexia. The cause of death in metaldehyde poisoning is often said to be respiratory failure (Booze and Oehme, 1985; Dolder, 2003), but this is generally not the case. Death may result from complications of prolonged seizure activity, such as pyrexia or disseminated intravascular coagulation; other signs reported in fatal cases include multi-organ failure, complications of aspiration or pulmonary oedema (Bates et al, 2012).
Temporary blindness is occasionally reported as a sequelae of metaldehyde toxicosis (Bishop, 1975; Bates et al, 2012) (Case report 1) and is probably because of seizure activity rather than a toxic effect of metaldehyde itself. Apparent memory loss has also been reported (Bishop, 1975).
The fatality rate in dogs with metaldehyde poisoning is approximately 14–17% (James, 1955; Studdert, 1985; Robertson et al, 1992; Yas-Natan et al, 2007; Bates et al, 2012).
Treatment
Treatment is recommended for ingestion of more than 2 mg/kg of metaldehyde by a dog (Dolder, 2003). This is equivalent to 66.7mg/kg bodyweight for a bait of 3% concentration (expressed as w/w).
If the dog is presented to the surgery within 1 hour of exposure and is asymptomatic then induction of emesis may be considered. Administration of an emetic after this time is best avoided because of the risk of seizures. Gastric lavage should be considered in cases of metaldehyde toxicosis, particularly if the dog is thought to have ingested a significant quantity, or has developed moderate to severe signs soon after ingestion. Activated charcoal is probably best avoided in conscious animals because of the risk of rapid-onset seizures. If gastric lavage is performed, activated charcoal can be left in the stomach after lavage.
The mainstay of treatment for dogs in metaldehyde toxicosis is controlling seizures and preventing the complications of prolonged seizure activity. Multiple drugs may be needed to control seizures. In a Veterinary Poisons Information Service (VPIS) review of cases, almost half the dogs requiring anticonvulsant therapy needed more than one sedative or anaesthetic (Bates et al, 2012). It is important to be prepared to escalate therapy quickly if the dog's condition seems to be deteriorating.
Diazepam is an appropriate first-choice drug to use in any animal with twitching or seizures, and may be considered for cases presenting with mild signs only. Propofol should be used immediately for any case presenting with moderate or severe signs. Animals may need to be on propofol for some time, so it is more practical to use a constant rate infusion. Midazolam or isoflurane can also be used.
In severe cases, barbiturates may also be considered as an alternative therapy. In the past (before agents such as propofol were available) this was one of the main methods of controlling seizures in dogs with metaldehyde toxicosis. Methocarbamol, a muscle relaxant that acts centrally, is also an option (Richardson et al, 2003) and can be used with diazepam or propofol.
Ideally, animals should be kept under anaesthetic for 6–12 hours and then the dose slowly tapered off and the animal observed closely to see whether seizures recur. If they do, then the dosage should be resumed.
Where possible, blood gases and body temperature should be monitored. Cooling measures should be employed for any animal with pyrexia, but a dog's temperature should fall once seizures are controlled. Acidosis is managed by restoring tissue perfusion through administration of intravenous fluids containing a bicarbonate precursor (such as lactate) or, if required, sodium bicarbonate therapy. Once other signs, such as seizures, are controlled, acidosis may resolve without the need for bicarbonate therapy.
Oxygen therapy may be required in animals with respiratory distress. Where possible, baseline liver enzyme activities should be measured when a dog is admitted and repeated at 24–48 hours, and beyond as required. These abnormalities usually resolve with supportive care alone and require no specific intervention.
Metaldehyde is not lipophilic (the log P is very low, only 0.12) and it is unlikely that lipid infusion would be useful. There are a few reports of using lipid emulsion in the management of metaldehyde toxicosis (Lelescu et al, 2017; Bergamini et al, 2020), but the animals in these reports received multiple therapies and it is impossible to determine the contribution of lipid emulsion to the successful outcome of the cases.
Haemodialysis has been shown to remove metaldehyde from canine plasma in vitro (Mauser et al, 2016) and is effective in clinical cases (Teichmann-Knorrn et al, 2020). Comparing 11 dogs with metaldehyde toxicosis treated with intermittent haemodialysis and 7 dogs managed conventionally, haemodialysis significantly decreased the requirement for anaesthesia and the length of hospitalisation. In addition, aspiration pneumonia occurred less often in dialysed patients (Teichmann-Knorrn et al, 2020).
Ferric phosphate
Ferric phosphate is available in some slug and snail killers, usually as blue-green pellets (Figure 3) in concentrations of 1% or 3%.
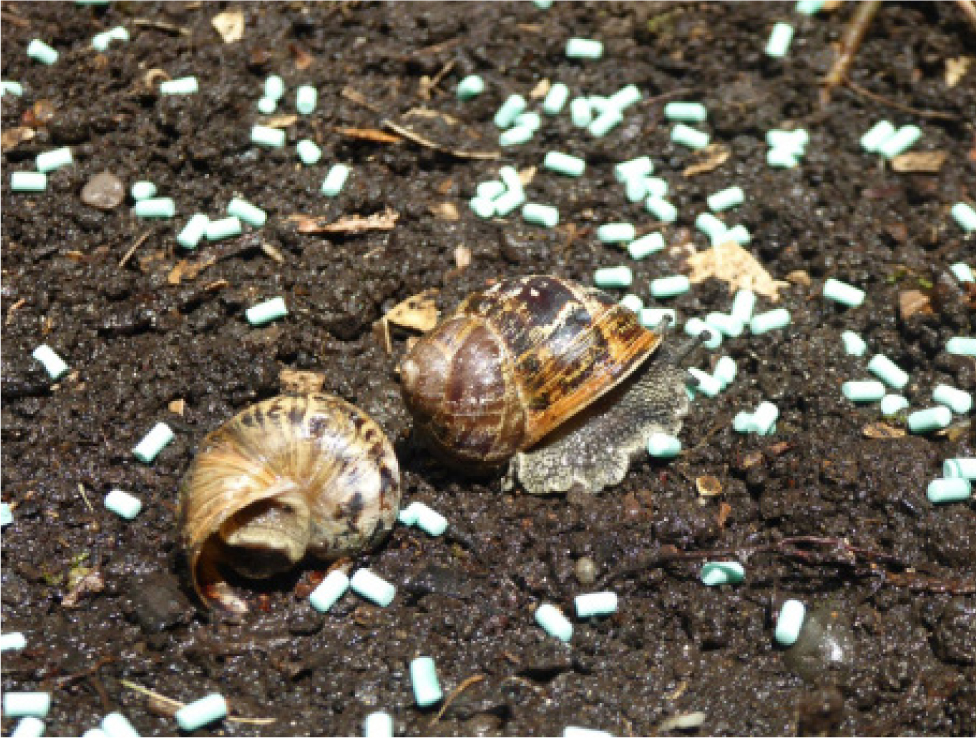
Case report 2. Ferric phosphate
A 9-year-old Labrador weighing 23kg ingested 300g of a slug bait containing 1% ferric phosphate. She developed vomiting (for 12 hours), diarrhoea, abdominal tenderness and was dehydrated. She was managed supportively and had recovered by 36 hours (VPIS case).
Some ferric phosphate products are described as containing hydrated ferric phosphate 12.5g/kg. This is still a 1% product concentration, because 12.5g of hydrated ferric phosphate is equivalent to 9.97g of anhydrous ferric phosphate (which is then rounded up to 10g/kg, or 1%).
Ferric phosphate is less toxic than ferrous salts of iron as it is practically insoluble in water and lipids, and has low oral bioavailability. In a review of cases of molluscicide ingestion reported to the National Pesticide Information Centre in America in a 10-year period, there were 215 cases involving ferric phosphate. Of these, 91% involved dogs and 39.5% (85 animals) developed clinical signs. In dogs, vomiting was the most common sign 71% (40/56) followed by diarrhoea (43%) and lethargy (25%). There were occasional reports of blood in the vomit (n=3) or diarrhoea (n=4). Other signs reported were anorexia, gastrointestinal distress, dehydration (Case report 2), fatigue, ataxia and one dog was reported to have had a seizure. There were no fatal cases (Buhl et al, 2013).
In a review of 37 cases of ferric phosphate molluscicide ingestion in dogs reported to the VPIS, 16 (43%) remained asymptomatic; the quantity ingested in most cases was unknown, but where reported it ranged from a few grams to 500g. Overall, 21 dogs (57%) developed signs. Signs reported were diarrhoea (n=11), vomiting (n=11), lethargy/depression/dullness (n=5), abdominal tenderness/pain (n=2), coloured stools (n=2), hyperaesthesia (n=2), ataxia (n=1), collapse (n=1), shaking (n=1), haematemesis (n=1), melaena (n=1) and leucocytosis (n=1). One animal developed liver damage with elevated liver enzymes and jaundice. Of the 21 dogs that developed symptoms, 20 recovered fully. Recovery time, where known (n=5), was 6–24 hours. The case of the dog that developed liver damage was ongoing at the time of follow up (Tizzard and Bates, 2018).
Mechanism of action
The early features of iron poisoning from ingesting soluble iron compounds result from the corrosive and vascular effects of iron, while the later effects are largely caused by the disruption of cellular processes. There may be severe gastrointestinal signs and the consequent fluid and blood loss may be substantial, leading to hypovolaemia and decreased cardiac output. This in turn results in tissue hypoxia, lactic acidosis and collapse circulatory system. Acidosis also occurs directly from the liberation of an unbuffered hydrogen ion when ferrous iron is converted to ferric iron.
Iron is rapidly cleared from the extracellular space by uptake into parenchymal cells, particularly in the liver. It causes mitochondrial damage, interfering with cellular respiration and resulting in cellular dysfunction, metabolic acidosis and, ultimately, cell death. Eventually, widespread organ damage becomes apparent, with the liver being particularly affected. Hepatic failure with hypoglycaemia and coagulopathy may develop and this is potentially fatal (Barr and Fraser, 1968; Gleason et al, 1979). These are the effects that occur in iron toxicosis following ingestion of soluble, bioavailable iron compounds found in iron supplements.
The toxic dose of iron is similar in humans and dogs (Brutlag et al, 2012). Toxic doses of elemental iron are:
- 5–20 mg/kg, mild clinically insignificant effects
- >20–60 mg/kg, moderate toxicity
- >60 mg/kg, severe toxicity
- >100–250 mg/kg, lethal toxicity (Reissman et al, 1955; Albretsen, 2006; Brutlag et al, 2012).
It is important to note that these doses are based on soluble bioavailable forms of iron and will greatly overestimate the toxicity of insoluble, poorly bioavailable iron compounds (Hall, 2013). Ferric orthophosphate has a relative bioavailability of only 6–32% (Hurrell, 1997) Studies in dogs found that iron salts varied in toxicity even when given in the same dose of elemental iron. Ferrous sulphate was the most toxic followed by the succinate, gluconate and then carbonate (D'Arcy and Howard, 1962). These ferrous compounds are bioavailable and used in pharmaceutical iron preparations.
Clinical effects
Slug and snail control products only contain 1–3% ferric phosphate and the other 99% or 97% is usually bran or wheat, and may cause gastrointestinal signs with diarrhoea, vomiting, lethargy, abdominal discomfort and coloured stools (Case report 2). Occasionally, haematemesis and melaena may occur (Buhl et al, 2013; Tizzard and Bates, 2018).
Iron poisoning is less of a risk with ferric phosphate-containing slug and snail killer, compared to iron-containing pharmaceuticals, because of the low oral bioavailability of iron in this form.
Treatment
The toxic doses of iron are based on soluble, bioavailable forms of iron and will greatly overestimate the toxicity of insoluble poorly bioavailable iron compounds (Hall, 2013). It is likely to be the other ingredients (eg bran, wheat) in these products that cause the gastrointestinal signs.
Iron is not adsorbed by activated charcoal and so the use of adsorbents is not recommended. Dogs that have ingested ferric phosphate molluscicide should receive symptomatic and supportive treatment, while gastrointestinal signs are managed.
Methiocarb
Methiocarb is a carbamate pesticide. It is used as an insecticide (often in seed dressings) but its use as a molluscicide was banned in the UK in 2015. Products may still be available, however. In summer 2021, two horses died after accessing an old cattle shed and eating from a sack of old slug bait (VPIS case data). The concentration in molluscicide pellets was usually 2, 3 or 4% methiocarb.
The LD50 of methiocarb in dogs is 10–25 mg/kg (Kimmerle, 1969; Lamb and Matzkanin, 1975). This is equivalent to 250–625 mg of a 4% bait per kg of bodyweight in dogs.
Mechanism of toxicity
Like organophosphates, carbamates bind to and competitively inhibit acetylcholinesterase. This results in accumulation of the neurotransmitter acetylcholine and activation of nicotinic receptors at skeletal muscle end plates, autonomic ganglia and within the central nervous system, and of muscarinic receptors at smooth muscle myoneural junctions and exocrine and endocrine secretory systems. Therefore, both nicotinic and muscarinic effects occur, although nicotinic receptors rapidly become desensitised. Myopathy is thought to be secondary to excessive acetylcholine stimulation of muscle.
Compared with the phosphorylated enzyme that results from organophosphate poisoning, the carbamylated acetylcholinesterase is more unstable, and spontaneous reversal to the carbamate and acetylcholinesterase occurs (Reiner, 1971). As a result, the effects of carbamate poisoning tend to be of much shorter duration compared with those of organophosphate poisoning and use of cholinesterase reactivators (such as pralidoxime) is unnecessary (Kurtz, 1990). Atropine acts as a noncompetitive antagonist by blocking the effect of the muscarinic receptors on target organs.
Clinical effects
The onset of effects from ingestion of methiocarb are rapid and usually occur within 30 minutes to 3 hours.
Mild-to-moderate effects of methiocarb poisoning include hypersalivation, vomiting, ataxia, diarrhoea, bronchial hypersecretion (Case report 3), constricted pupils, muscle fasciculation, tremors and twitching, weakness, shaking, hyperaesthesia, pyrexia, restlessness and urinary incontinence (Firth, 1992). Tachycardia can develop later. Collapse, bradycardia, respiratory depression, convulsions, cyanosis and coma may occur in severe cases.
Laboratory findings are generally nonspecific in carbamate poisoning and include increased packed cell volume, and raised serum aspartate transaminase and creatine kinase. Elevated serum amylase may be noted. Cholinesterase activity will be decreased. A cholinesterase activity of more than 50% of normal is suspicious and an activity of less than 25% of normal is diagnostic (Means, 2013).
Case report 3. Methiocarb
A one-year-old female Labrador weighing 29kg presented 1 hour after ingestion of slug bait containing 3% methiocarb. The incident occurred after an old barn was cleared and the dog accessed the container of pellets which had reportedly been placed in there years previously. The dog developed hypersalivation, nystagmus and severe diarrhoea, had respiratory distress and was difficult to intubate. A volume of mucous and fluid drained out of the airway when the dog's head was dangled over the edge of the consult table. A gastric lavage was performed and green-tinged material was retrieved. The animal progressed to twitching and then seizures with severe bradycardia (12 bpm) and cyanosis. The dog was managed with diazepam, propofol and isoflurane, intravenous fluids and activated charcoal. Recovery was seen 12 hours after ingestion. Blood tests showed raised alanine transaminase and amylase and these had resolved by 96 hours (VPIS case).
Recovery may take 1–2 days because of the slow-release nature of the pellets. However, a study of 56 dogs with methiocarb poisoning showed that 90% were discharged in 12 hours or less (Firth, 1992).
Sequelae can occasionally occur after methiocarb toxicosis in dogs. Intermediate syndrome is a potential sequelae of carbamate or organophosphate insecticide toxicosis, but few cases have been reported in animals (Tinson et al, 2017). The syndrome generally occurs 24–72 hours after onset of acute signs and may last 7–14 days or longer (Gualtieri, 2011). Typical signs are neuromuscular weakness of the thoracic limb, neck and respiratory muscles (Case report 3). There may also be cranial nerve deficits, anorexia, diarrhoea, muscle tremors, constricted or dilated pupils, depression and convulsions (Gualtieri, 2011). Intussusception has also been reported in dogs with methiocarb poisoning (Corfield et al, 2008; VPIS case data).
Treatment
An emetic could be considered if ingestion of methiocarb was very recent and the animal appears asymptomatic. Otherwise, a gastric lavage could be considered, particularly if a large quantity has been ingested and emesis is contraindicated (because of rapid-onset neurological signs).
Atropine is the main drug used in the management of carbamate poisoning. It should be given to reverse cholinergic effects and control bradycardia and bronchial secretions (Means, 2013). Large doses may be required for effect. The dose is 0.2–0.5 mg/kg, and should be repeated as required. In mild to moderate cases, it can be given by intramuscular or subcutaneous injection. In severe cases, about one-third to one quarter is given by slow intravenous injection (as a 2% solution) and the rest is given by subcutaneous injection. Tachycardia is not a contraindication to initial atropine use. Further doses can be lower and based on clinical signs, including heart rate (Means, 2013). It should be noted that atropine has no effect on nicotinic receptors and will not counteract skeletal muscle tremors, weakness or neuromuscular paralysis.
Further management of animals with methiocarb toxicosis is supportive. The heart rate, temperature and bronchial secretions should be monitored. Oxygen can be given for respiratory depression, with ventilation, if required. If sedation is required, diazepam or a barbiturate may be given.
Phenothiazine sedatives (eg acepromazine) and succinylcholine should be avoided in animals with carbamate poisoning because they have neuromuscular blocking activity and will exacerbate the toxic effects of methiocarb (Platt and Garosi, 2012). Antibiotics such as aminoglycosides (eg streptomycin, gentamicin, neomycin), polypeptides (eg polymixin A and B) and clindamycin should also be avoided since they may also have neuromuscular blocking effects (Lee et al, 1977; Paradelis et al, 1980; al Ahdal and Bevan, 1995).
Dogs with the intermediate syndrome following methiocarb toxicosis should be managed according to their symptoms and the support they need.
Slug and snail deterrents
In addition to products that kill snail and slugs, other products are available which deter these pests. These products usually contain diatomaceous earth or wool.
Diatomaceous earth
Diatomaceous earth is a naturally occurring, soft, siliceous sedimentary rock consisting of the fossilised remains of diatoms, a type of hard-shelled algae. It is used in some slug/snail deterrents, as a mite treatment and in cat litter. Most cases of exposure in pets relate to usage as a flea treatment rather than as a slug deterrent.
Diatomaceous earth is abrasive to snails and slugs and acts as a deterrent. It is of low toxicity, and it is generally regarded as safe (GRAS) by the United States Food and Drug Administration (FDA, 2015). Ingestion may cause mild gastrointestinal signs, but inhalation is more of an issue and can cause cough and respiratory distress as would be expected to result from any particulate dust. Heavy exposure may result in more significant respiratory signs. Respiratory distress, bilateral harsh breathing sounds, laboured respiration, respiratory arrest and death was reported in a cat after diatomaceous earth was applied all over the house and garden (VPIS case data). On the skin, diatomaceous earth may cause irritation and dryness. Repeated exposure may cause dermatitis and severe skin irritation (VPIS case data).
Management of exposure to diatomaceous earth is supportive. Gut decontamination is not required, and pets should be monitored for respiratory signs. Pets with dermal contamination should be washed.
Wool pellets
The pellets are made from sheep wool, applied to the soil around the plant and water added. Wool fibres have very fine scales with small barbs and the addition of water makes the pellets swell and lock together to form a protective mat. The wool fibres are very hygroscopic and this, plus the sand and grit already in the wool and the potassium salts from the sweat glands of the sheep, reportedly absorb some of the slime from the slug or snail's foot to cause irritation (Vitax Ltd, 2021). These properties physically deter slugs and snails.
Wool pellets are not a toxicological risk but if eaten dried they could swell in the gut and cause an obstruction. Other possible signs include vomiting, thirst, constipation, and possibly abdominal discomfort and distention. Dogs who have ingested these pellets should be managed supportively. Owners should be advised to observe for signs of obstruction and return if required.
Conclusions
Several different substances are used in the control of slugs and snails. Metaldehyde was commonly used but this has been banned in the UK from March 2022, although it may take some years before metaldehyde poisoning becomes uncommon. Methiocarb was banned in 2015 but poisoning cases still occur. Both metaldehyde and methiocarb can cause rapid-onset seizures and severe poisoning. Most baits contain ferric phosphate, which is of comparatively low toxicity as this iron compound has low bioavailability. Slug and snail deterrents are becoming increasingly available and typically contain diatomaceous earth or wool. ingestion. Blood tests showed raised alanine transaminase and amylase and these had resolved by 96 hours (VPIS case).
KEY POINTS
- Methiocarb slug baits were withdrawn in the UK in 2015; outdoor use of metaldehyde has been banned since March 2022. Both compounds can cause serious poisoning in pets.
- Ferric phosphate is now commonly used in slug baits but the iron in this compound is of low oral bioavailability and clinical signs after ingestion are generally limited to gastrointestinal effects.
- New products for control of slugs and snail are deterrents containing wool pellets or diatomaceous earth. These are generally of low toxicity but animals should be discouraged from eating them.