Immune-mediated haemolytic anaemia (IMHA) is a frequent cause of anaemia and is one of the most common autoimmune conditions diagnosed in dogs (Goggs et al, 2015). In cats, IMHA is an uncommon cause of anaemia (Swann et al, 2016), with disorders such as infections and neoplasia appearing to be more prevalent causes (Korman et al, 2013). However, IMHA in cats can still result in a severe and life-threatening condition (Swann et al, 2016).
Generally, IMHA has been categorised into primary (idiopathic) or secondary. The American College of Veterinary Internal Medicine (ACVIM) consensus statement on the diagnosis of IMHA in dogs and cats (Garden et al, 2019) proposed the designation of ‘non-associative’ for ‘primary’ and ‘associative’ for ‘secondary’ IMHA. The rationale behind this suggested terminology is that the term ‘primary’ would imply all triggers to have been definitively ruled out, while ‘secondary’ would indicate a proven causality between concurrent pathologies. In practice many cases may be ambiguous, so the term ‘associative’ describes cases of IMHA in which one or more comorbidities are identified, whereas ‘non-associative’ implies that no other disease has been identified with the diagnostic tests used.
Non-associative or primary IMHA is the most common form of IMHA in both dogs and cats (Swann et al, 2016). It typically affects young adults to middle aged animals (Piek et al, 2008), and some dog breeds, such as Cocker Spaniels, English Springer Spaniels, Poodles and Old English Sheepdogs, appear to be overrepresented (Miller et al, 2004).
Associative or secondary IMHA occurs when an underlying disease (such as neoplasia or infections), or exposure to exogenous substances (including drugs, toxins and vaccines) accompanies the presence of antibodies targeting epitopes of red blood cells (Piek et al, 2008). In some cases the anaemia is responsible for initial presentation, while in others IMHA can develop when the animal is already being treated for another condition, such as pancreatitis, and this can be responsible for a sudden deterioration.
Regardless of its classification, animals with IMHA usually present with clinical signs including lethargy, inappetence, pigmenturia, tachycardia, pale mucous membranes and fever (Piek et al, 2008). Diagnosis is based on the demonstration of haemolysis and red blood cell-bound immunoglobulins (Piek et al, 2008) in the anaemic animal.
Treatment differs between cases of non-associative (primary) and associative (secondary) IMHA. While the essential therapeutic aim in non-associative IMHA is to control the aberrant immune response, treatment for secondary IMHA should be directed towards the putative underlying cause, and immune modulation may or may not be required.
Currently, IMHA remains a potentially fatal disease, with reported mortality rates for primary/non-associative IMHA ranging from 29–70% in dogs (Klag et al, 1993; Piek, 2011) and between 24–40% in cats (Kohn et al, 2006; Swann et al, 2016).
Pathogenesis
Normally, canine red blood cells have a life span of 100–120 days (McCullough, 2003) while feline red blood cells remain in circulation for approximately 72 days (Eibert and Lewis, 1997). Once senescent, red blood cells are removed from the circulation via the mononuclear phagocytic system in the liver and spleen (McCullough, 2003). IMHA is characterised by premature destruction of red blood cells (McCullough, 2003).
Specifically, IMHA consists of a type II hypersensitivity reaction where red blood cells become coated with antibodies, complement or both. This leads to erythrocyte destruction either by direct lysis (intravascular haemolysis) or by enhanced mononuclear phagocytic system uptake (extravascular) (Figure 1).
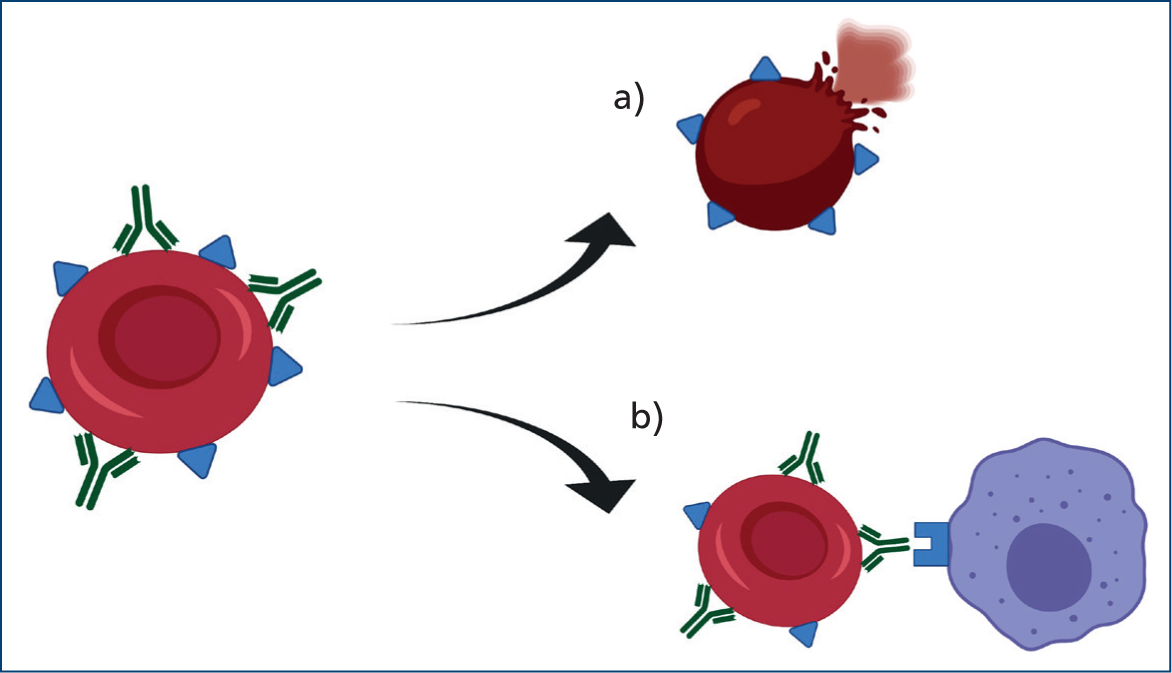
The degree and site of haemolysis depend on the type and load of immunoglobulins and complement cascade activation. Both immunoglobulin G (IgG) and M (IgM) have been detected on the surface of red blood cells in dogs and cats with IMHA (Morley et al, 2008). IgG-mediated destruction seems to be the most frequent mechanism involved in dogs (Harkin et al, 2012), and IgM have been isolated more frequently in cats (Kohn et al, 2006).
IgG are monomeric immunoglobulins (Figure 2) that cause haemagglutination at very high concentrations (Harkin et al, 2012). Thus, autoagglutination is not a common feature for IgG-coated red blood cells.
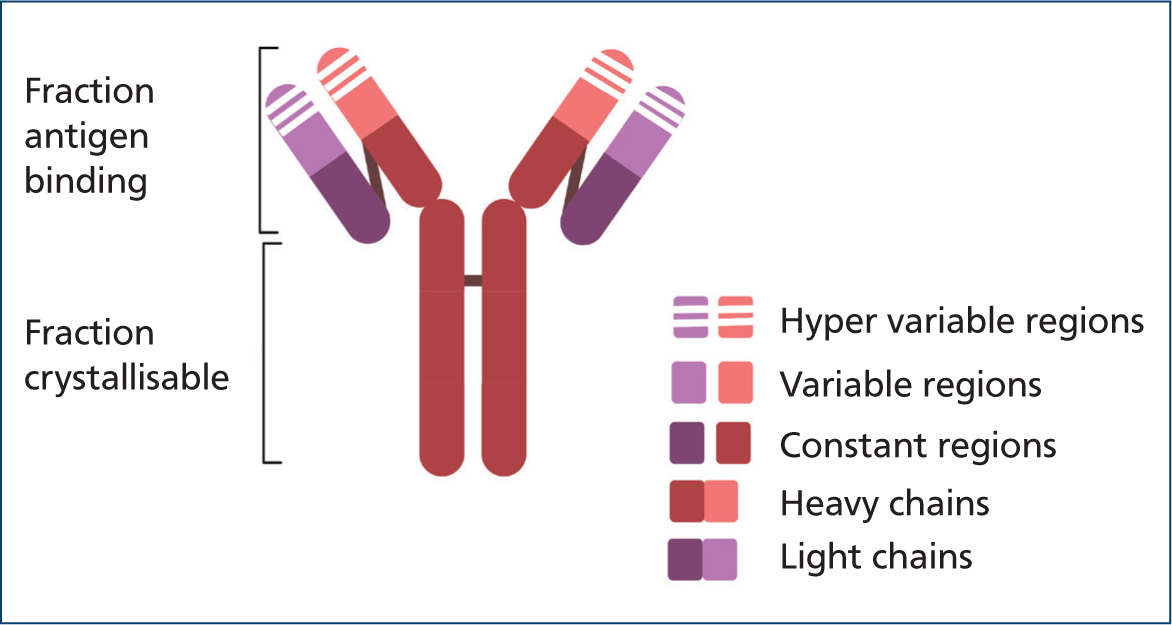
IgG mainly cause extravascular haemolysis via the mononuclear phagocytic system, which recognises and binds IgG's crystallisable fragment region (McCullough, 2003) as shown in Figure 3.
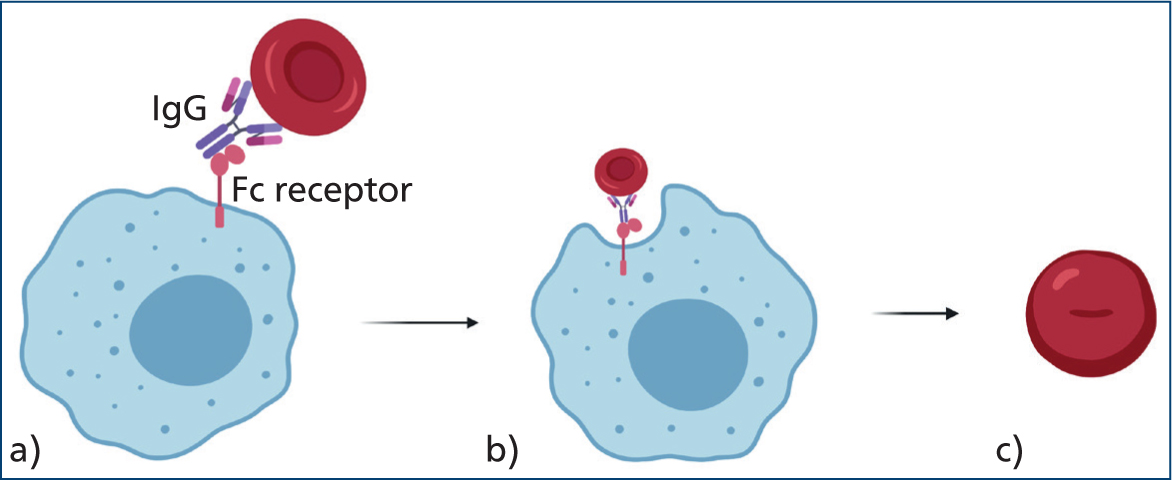
IgM are pentameric immunoglobulins that can easily promote haemagglutination (Figure 4) and associated significant intravascular, albeit mainly extravascular haemolysis.
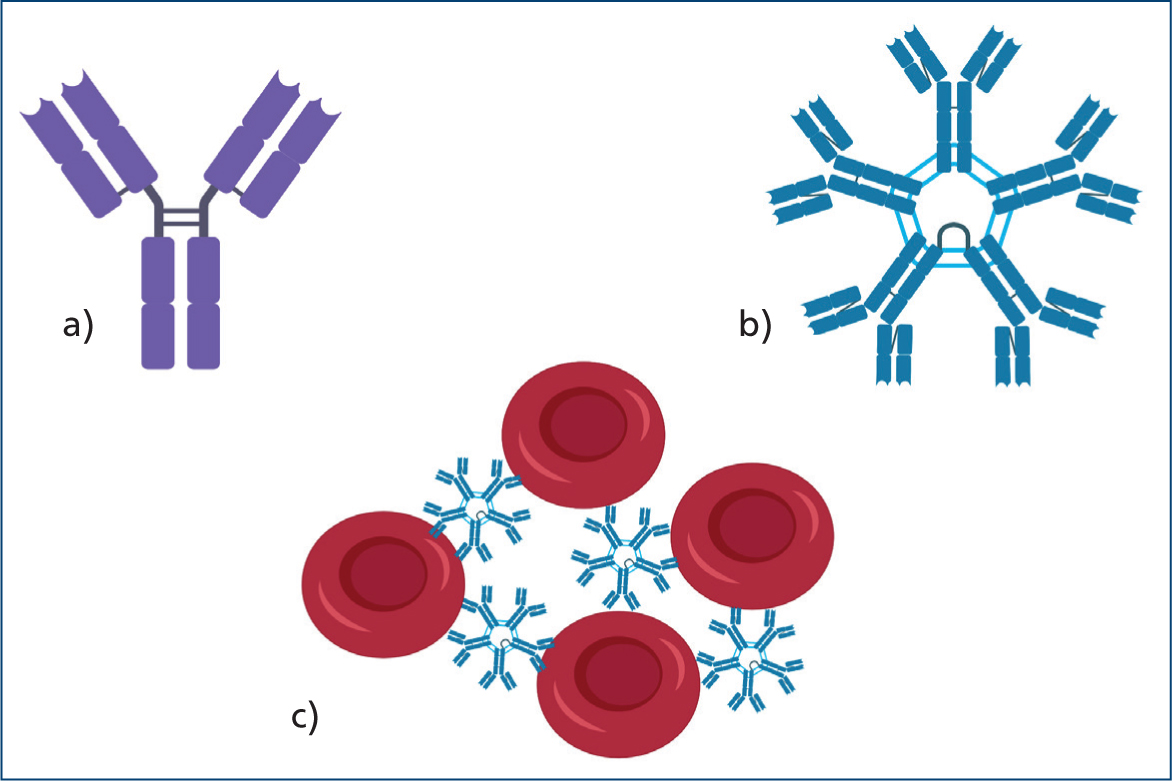
IgM-coated red blood cells can also contribute to complement activation (Harkin et al, 2012). The membrane attack complex causes immediate intravascular lysis, creating holes in the red blood cell membrane and allowing uncontrolled influx of water and electrolytes into the cell, which subsequently swells and ruptures (McCullough, 2003). The C3b-membrane complex (opsonin) can be recognised by splenic macrophages, leading to partial phagocytosis and the production of circulating spherocytes (McCullough, 2003) (Figure 5). Spherocytes are ultimately lysed within the spleen or liver because of their increased rigidity.
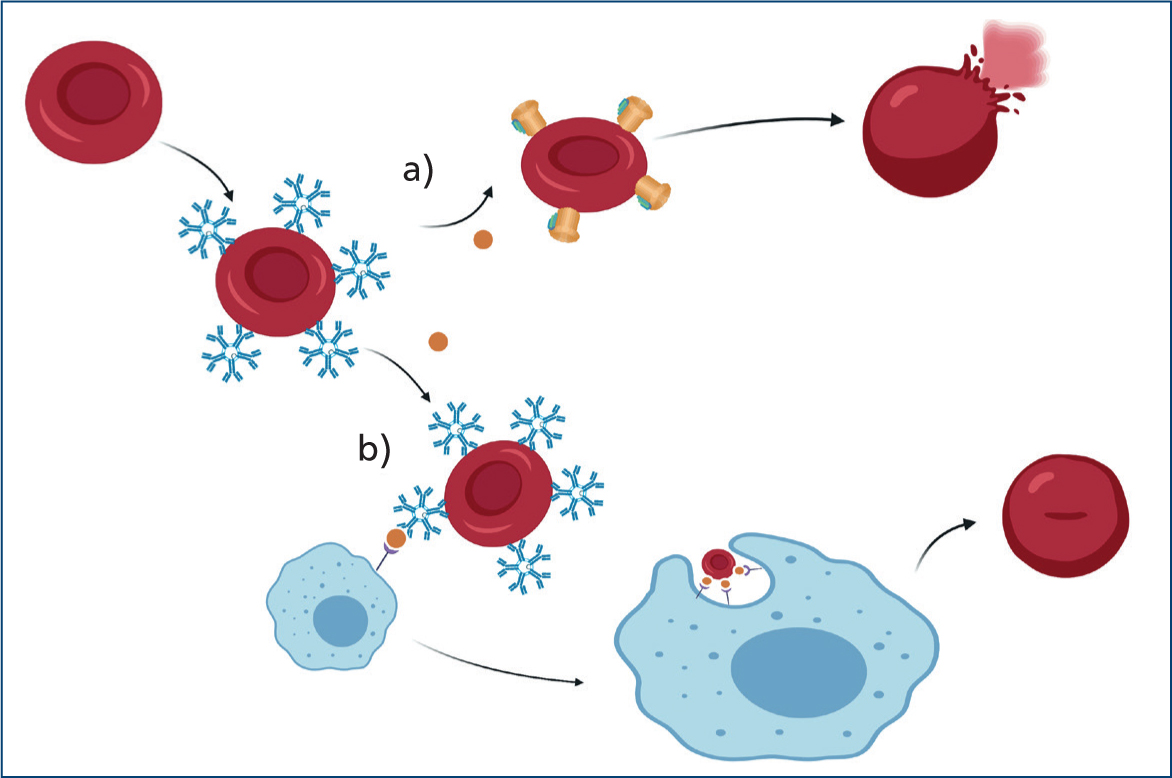
Signalment
Primary or non-associative IMHA presents in young to middle aged dogs with a median age of 6 years (Piek, 2011). Female dogs appear to be overrepresented (Miller et al, 2004), and the effect of the neuter status remains controversial (Carr et al, 2002; McCullough, 2003; Weinkle et al, 2005; Goggs et al, 2015; Sundburg et al, 2016). Pregnancy-associated IMHA is a well-known occurrence in humans (Piatek et al, 2015) but, to the authors' knowledge, presumed cases have so far been reported only in one pregnant dog (Fernandez et al, 2020) and one pregnant cat (Kopke et al, 2019).
Cocker Spaniels have been consistently recognised as an overrepresented breed; one study reported this breed to be 12.2 times more likely to be diagnosed with IMHA than any other breed (Miller et al, 2004). An Australian study reported Maltese Terrier dogs, Springer Spaniels, Hungarian Vizslas and Airedale Terriers to be overrepresented (McAlees, 2010), while a US-based article reported IMHA to be significantly more prevalent in Poodles, Miniature Schnauzers and Collies (Reimer et al, 1999). These diverging data might suggest that geographical location and exposure to different environments or different breed lines could also play a role in the reported prevalence of IMHA among different dog breeds.
Canine blood types have also been investigated, and dog erythrocyte antigen 7 positivity has been suggested to have a protective effect in Cocker Spaniels in one study (Miller et al, 2004). However, only 10 Cocker Spaniels with IMHA were recruited in that study.
In cats, secondary or associative IMHA was previously thought to be more common than primary IMHA (Scott et al, 1973; Werner and Gorman, 1984). However, a more recent study involving 107 cats reported that a minority of cats with IMHA (32.7%) were diagnosed with a concurrent putative cause (Swann et al, 2016). The same study showed that cats between 2 and 6 years old were twice as likely to develop primary IMHA. Male cats were previously believed to be overrepresented (Kohn et al, 2006), but this was not supported by Swann et al (2016). No feline breed predisposition has been identified so far (Swann et al, 2016).
Clinical presentation
Most animals with IMHA present with clinical signs attributable to anaemia and tissue hypoxia (McCullough, 2003). Common physical exam findings include pale mucous membranes, tachycardia, tachypnoea, hyperdynamic peripheral pulses and systolic murmurs. However, a minority of cases have a much slower disease course, and may have non-specific signs like anorexia, lethargy, a slowly developing exercise intolerance and weakness, which might be accompanied by icterus and discolouration of urine and faeces (Piek, 2011). Fever and concurrent gastrointestinal signs are reported in 46% and 30% of dogs, respectively (Piek et al, 2008).
Icterus in cats with IMHA appears to be uncommon, with an incidence of 10% (Kohn et al, 2006).
A minority of dogs presents with clinical signs secondary to thrombocytopenia (petecchiae, ecchymosis, epistaxis, melaena) (Orcutt et al, 2010), and this is less common in cats (Kohn et al, 2006).
Diagnosis
Demonstration of anaemia
This is more reliably done with a manual packed cell volume, rather than with a calculated haematocrit, especially if agglutination is present (Zandecki et al, 2007). In some cases the reference values vary; neonates (O'Brien et al, 2014) and pregnant dogs (Allard et al, 1989; Kimura and Kotani, 2018) normally have lower packed cell volume; while some sighthounds (Zaldívar-López et al, 2011) and Dachshunds (Torres et al, 2014) have higher red blood cells counts.
Assessment of regeneration
Reticulocytes are anucleated immature red blood cells which still contain ribonucleic acid (RNA). They can be identified in a blood smear with new methylene blue stains (which precipitate the RNA, highlighting it as cytoplasmatic blue clumps). Reticulocytosis is an increase in the number of circulating reticulocytes above the reference interval, which reflects a physiological response of the bone marrow to persistent renal cortical hypoxia (Nitsche, 2004), and subsequent elevated erythropoietin concentration (Cowgill et al, 2003), leading to increased proliferation and differentiation of erythroid precursors.
Reticulocyte count is the most accurate means of quantifying the degree of regeneration (Church, 2017). A reticulocyte percentage above the reference interval is interpreted as regeneration. However, reticulocyte percentage may not be useful to assess if the degree of regeneration is adequate as it does not consider the severity of anaemia. Means of assessing the adequacy of the degree of regeneration include the absolute reticulocyte count, which is provided by many haematology analysers or may be calculated. This can be done by calculating reticulocyte % × red blood cell count (millions/µl)×10, or the corrected reticulocyte percentage: reticulocyte % × animal's haematocrit or packed cell volume ÷ ‘normal’ haematocrit or packed cell volume (Cowgill et al, 2003).
In cats there are two types of reticulocytes. Aggregate reticulocytes are reliable indicators of recent regeneration (appearing in circulation after approximately 48 hours) and are the only type that should be included in the reticulocyte count. Aggregate reticulocytes develop into punctate reticulocytes with time, and can remain in circulation for 14–21 days, so they should not be considered as a sign of recent regeneration (Paltrinieri et al, 2018). Feline aggregate and punctate reticulocytes are shown in Figure 6.
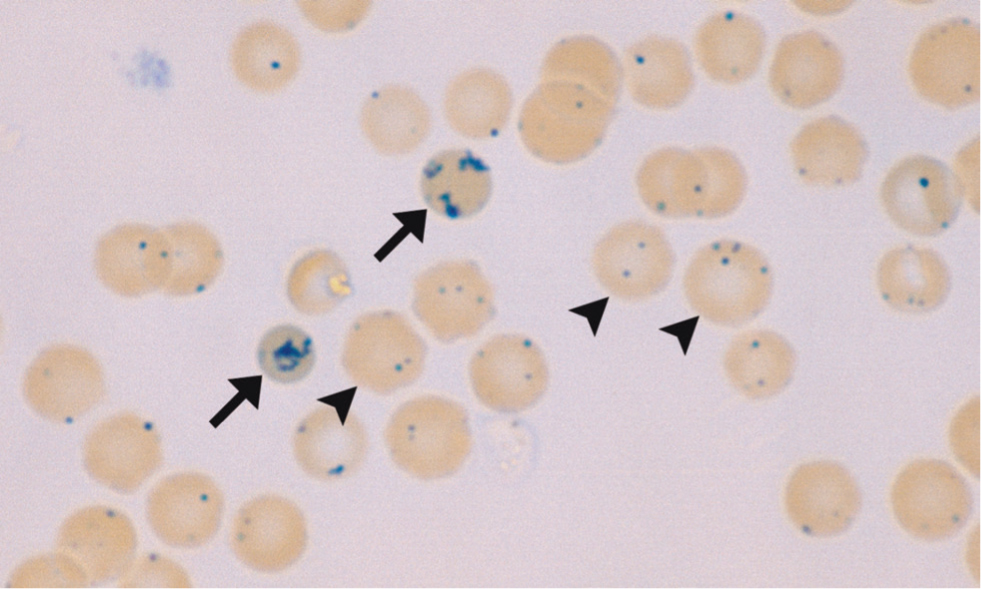
Reticulocytes can also be observed as polychromatophils on films stained with Romanowsky-type stains (such as Diff-Quik), which appear more intensely blue stained and larger than mature red blood cells. In one study, polychromasia was deemed the most reliable regenerative sign in dogs, when reticulocyte count was not available (Hodges and Christopher, 2011).
Other indicators of regeneration on smear include macrocytosis (Hodges and Christopher, 2011), Howell–Jolly bodies and nucleated red blood cells (Cowgill et al, 2003), as shown in Figure 7.
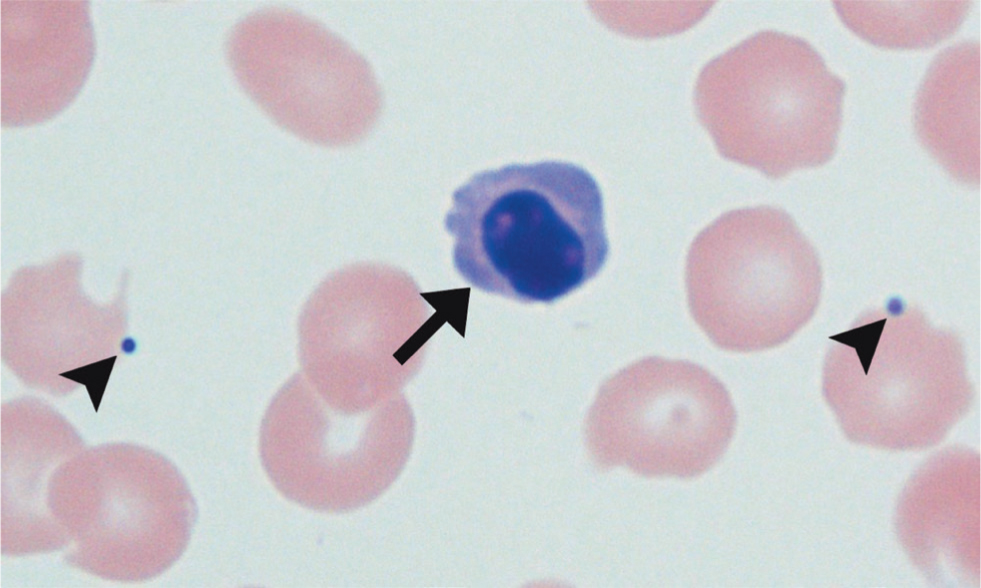
One third of dogs, and even more cats, with IMHA may lack signs of regeneration on presentation (McCullough, 2003; Piek, 2011; Swann et al, 2016). This may be the result of a pre-regenerative state, as it can take 2–5 days for the bone marrow to mount a regenerative response (Grimes and Fry, 2015). Alternatively, in cases in which the regenerative response appears poor after a few days, consideration should be given to bone marrow affection, including precursor-mediated IMHA (non-regenerative IMHA or precursor immune-mediated anaemia), marrow damage secondary to hypoxaemia, or coexisting bone marrow disease. In these animals, investigation of concurrent bone marrow pathologies should be considered. Weiss (2008) described bone marrow involvement associated with non-regenerative IMHA and concluded that multiple mechanisms may contribute to the lack of erythroid regeneration. Some cases of non-regenerative IMHA are caused by the immune-mediated destruction of bone marrow erythroid precursor cells (pure red cell aplasia), while others may show a variety of intrinsic bone marrow changes including dysmyelopoiesis, myelonecrosis and myelofibrosis. The latter population frequently presents with concurrent neutropaenia and thrombocytopenia, and the 60-day survival rate appears to be lower (Swann and Skelly, 2016).
It is fair to conclude that immune-mediated anaemias are a spectrum of related disorders, ranging from pure red cell aplasia, in which the immune system targets early erythroid precursors, to regenerative IMHA in which the target is mature circulating erythrocytes. However, these may occur concurrently in the same animal (Assenmacher et al, 2019).
If a regenerative response has been documented, blood loss should be excluded. Figure 8 illustrates a suggested approach to the animal with regenerative anaemia. It must be noted that while in the majority of cases only one pathophysiological mechanism may be involved, there are some exceptions where features of haemorrhage overlap with haemolysis, such as in cases of ruptured haemangiosarcomas. It is also worth noting that animals with chronic blood loss frequently present with poor (if any) signs of regeneration.
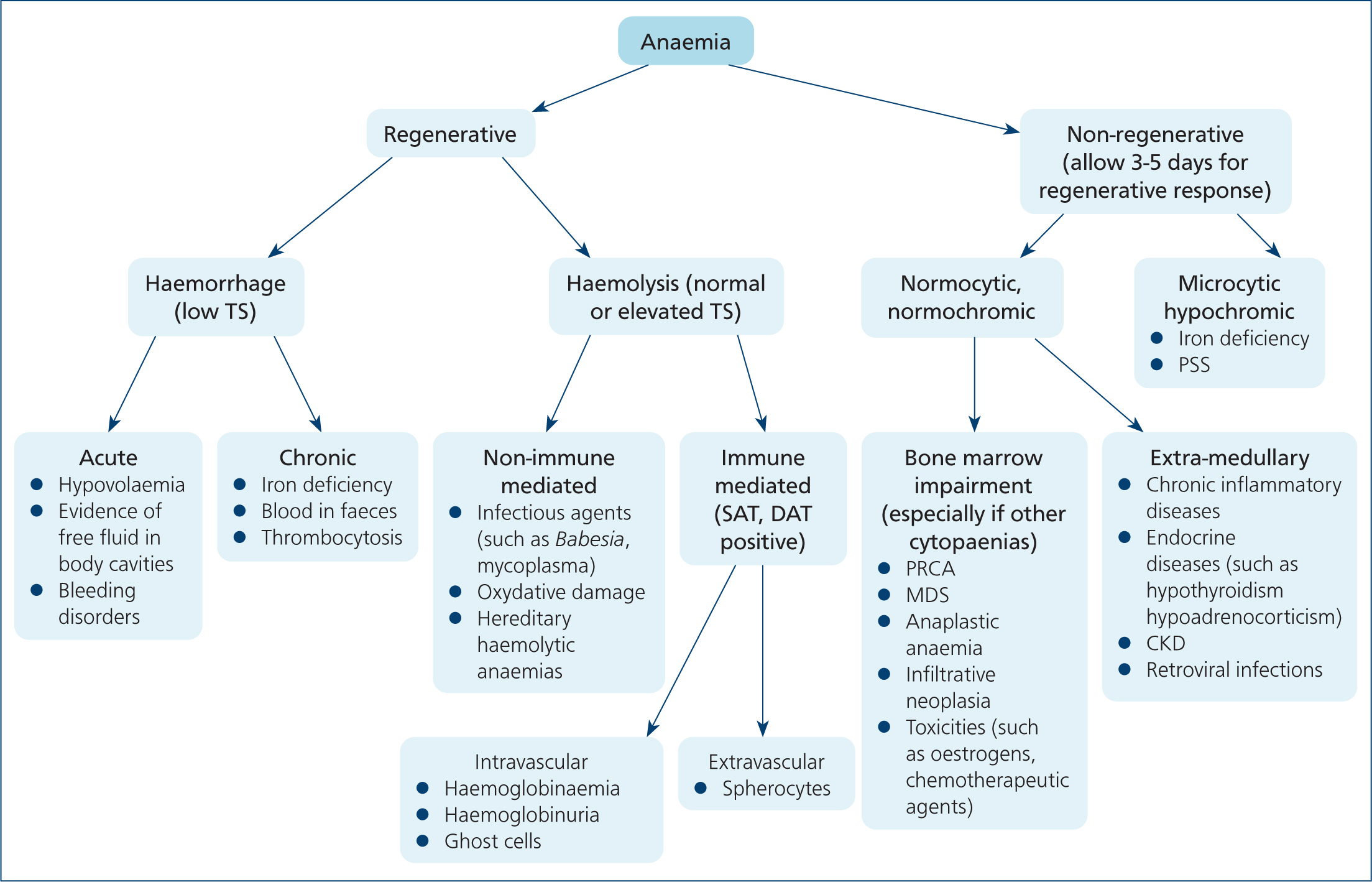
Signs of haemolysis
Once blood loss has been excluded, signs of haemolysis should be determined to further support management of IMHA.
Spherocytes (Figure 9) can be observed on blood smears as small, round, intensively stained red blood cells lacking the typical central pallor (Balch and Mackin, 2007). Spherocytes are considered to be evidence of extravascular immune-mediated erythrolysis, as they are the result of partial phagocytosis.
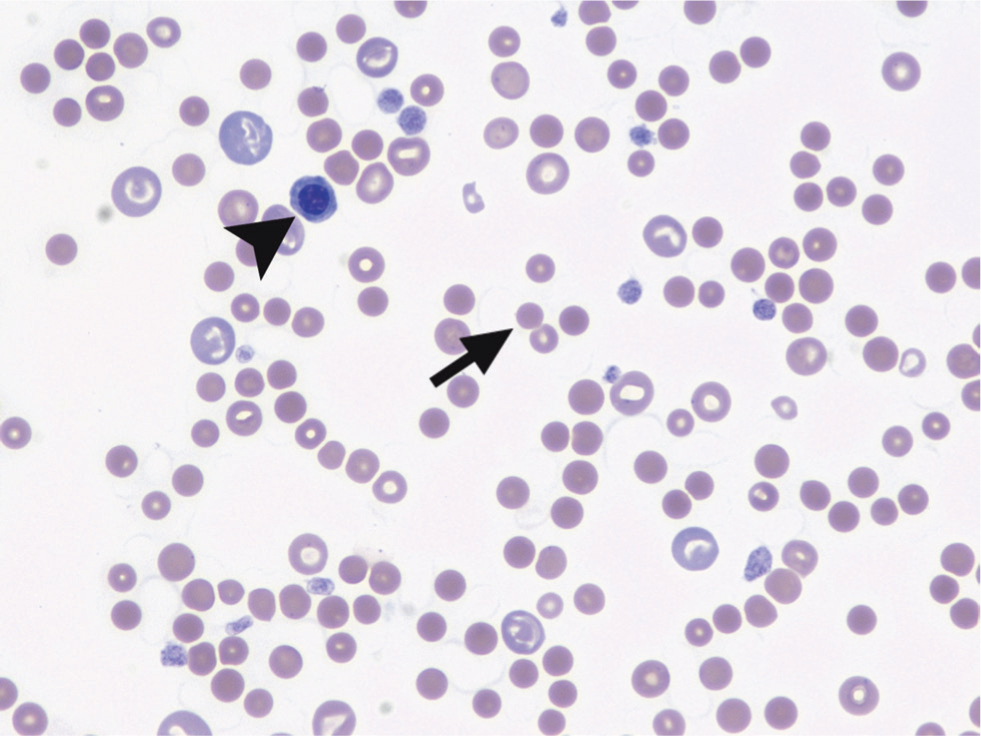
Five or more spherocytes per high power field showed a sensitivity of 63% and a specificity of 95% for the diagnosis of IMHA, compared to a lower cut-off value of ≥3 spherocytes per high power field (sensitivity of 74%, specificity of 81%) (Paes et al, 2013).
Although typical, spherocytes are not pathognomonic for IMHA as they can also be found in conditions like snake envenomation (Lenske et al, 2018), bee stings (Nair et al, 2019) and inherited red blood cell enzymatic deficiencies (Slappendel et al, 2005).
Spherocytes are not reliably identified in feline smears because normal red blood cells are smaller and lack a well-defined central pallor (McCullough, 2003). As a result, the osmotic fragility test has been proposed as an additional tool to diagnose IMHA in cats (Kohn et al, 2006). An increased osmotic fragility is not pathognomonic of IMHA, but may support it. Osmotic fragility may also be abnormal in pyruvate kinase and spectrin deficiency, azotaemia, zinc intoxication (Slappendel, 1998; MacNeill et al, 2019), hypophosphataemia (Adams et al, 1993), infectious and inflammatory diseases (Ottenjann et al, 2006).
Ghost cells are considered a sign of intravascular haemolysis, as they result from partially lysed erythrocytes that retain their basic cytoskeletal structure (Swann et al, 2016), but these may represent an in-vitro artefact in samples not promptly examined (MacNeill et al, 2019).
Urinalysis may show bilirubinuria and/or haemoglobinuria. In dogs, mild bilirubinuria can be a normal finding, especially in concentrated samples from male dogs (Piech and Wycislo, 2019).
Haemoglobinuria occurs when there is excess free haemoglobin in plasma after intravascular haemolysis (Piech and Wycislo, 2019).
With extravascular haemolysis, erythrocytes are degraded within macrophages and free haemoglobin is not released into the circulation. Hence, haemoglobinaemia or haemoglobinuria do not occur, unless concurrent intravascular haemolysis is present (Tasker, 2012).
In some cases, haemoglobin oxidises, becoming methaemoglobin and leading to brown discoloured urine (Piech and Wycislo, 2019).
Demonstration of an immune-mediated component
Although most cases of haemolysis in dogs and cats are immunemediated, there are instances in which it is not (Fleishman, 2012). Enzymatic abnormalities, like phosphofructokinase or pyruvate kinase deficiencies, that compromise the integrity and resilience of red blood cells have been reported in both dogs and cats (Owen and Harvey, 2012), as well as severe oxidative damage to red blood cells, secondary to zinc (Volmer et al, 2004; Blundell and Adam, 2013) or onion (Tang et al, 2008) toxicity. Additionally, mechanic microangiopathic trauma to red blood cells can occur in cases of haemangiosarcoma (Hammer et al, 1991).
The saline agglutination test is a readily available test that can be performed in-house (Figure 10). If false positives are suspected (such as high total proteins or fibrinogen serum concentration), repeating the test, after washing erythrocytes three times in a 1:4 ratio with saline, is recommended (Caviezel et al, 2014). The test should be performed at 37°C, as erythrocytes from normal dogs have been reported to spontaneously agglutinate at lower temperatures (Garden et al, 2019).
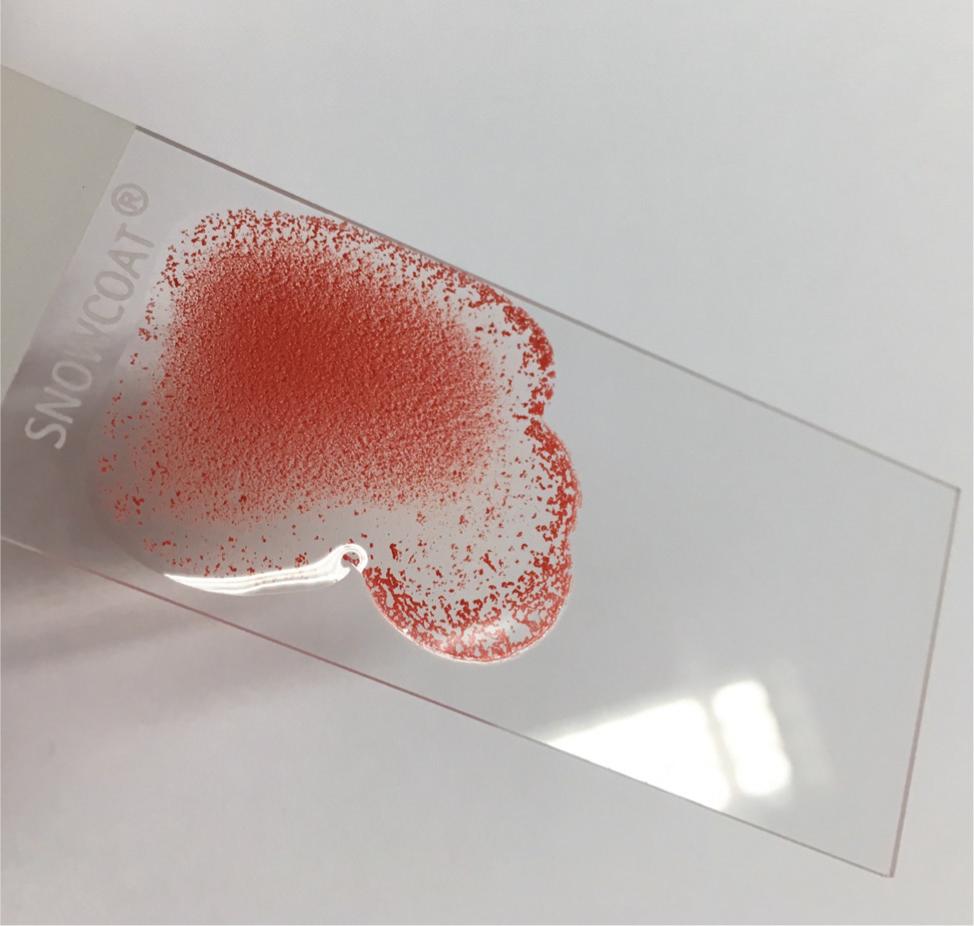
The Coombs test, or direct antiglobulin test, uses species-specific antibodies to detect immunoglobin and/or complement bound to erythrocytes (MacNeill et al, 2019). Increasing dilutions of polyvalent or monovalent antibodies are incubated with the animal's red blood cells, and the test is considered positive when agglutination occurs (MacNeill et al, 2019) as shown in Figure 11. Specific laboratories may consider low positive Coombs titres (1:2) to be of unclear significance, although there is no reliable evidence to support this. Results should be interpreted with caution, and communication with the laboratory is recommended. As for any test result that does not correlate with the clinical picture, repeating the test must be considered to try to reduce the possibility of spurious results.
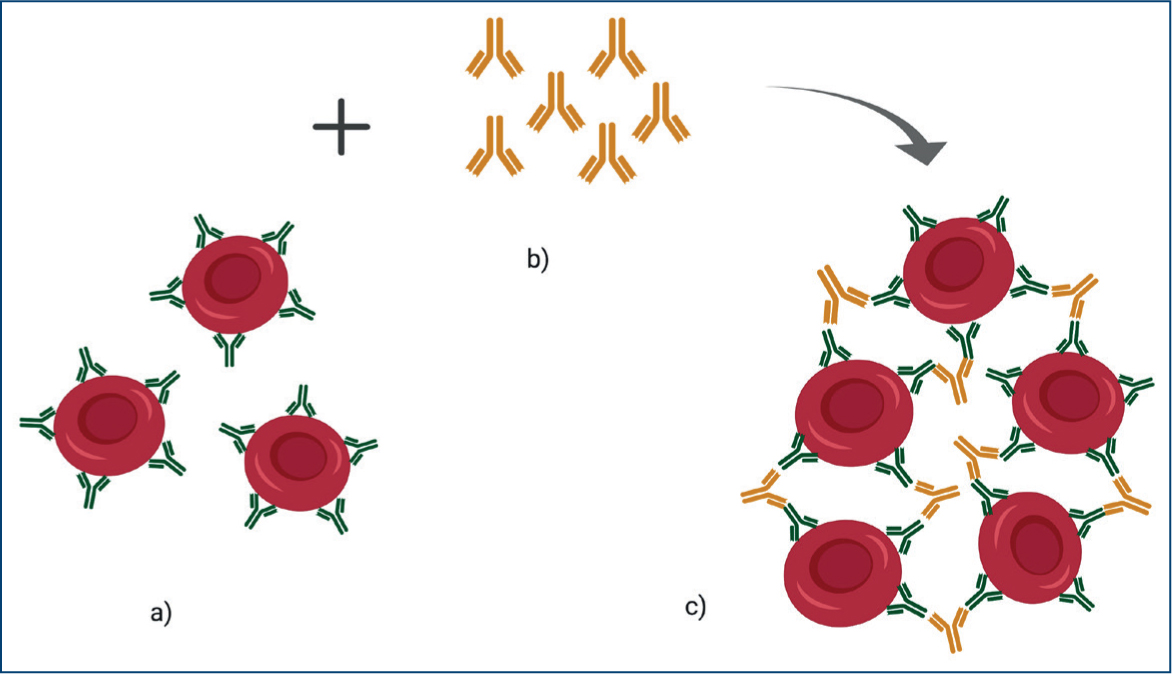
Reported Coombs test sensitivities are between 37% (Reimer et al, 1999) and 77% (Carr et al, 2002; Weinkle et al, 2005) in dogs and 82% in cats (Tasker et al, 2010), while specificity ranges between 94 and 100% in dogs (Overmann et al, 2007; Paes et al, 2013; Caviezel et al, 2014) and 95 and 100% in cats (Kohn et al, 2006; Tritschler et al, 2016). Coombs test accuracy is thought to be impaired by immunosuppressants, blood transfusions and by prolonged blood sample storage. However, one study demonstrated that dogs with IMHA, treated with immunosuppressants, remained positive until clinical remission was achieved; blood transfusions received 1–21 days before testing did not affect direct antiglobulin test status, and preserving ethylenediaminetetraacetic acid samples at 4°C did not affect results (Caviezel et al, 2014).
Flow cytometry can also be applied to detect immunoglobulins bound to red blood cells. In dogs, the test was reported to be 100% sensitive, 87.5% specific (Wilkerson et al, 2000) and have positive and negative predictive values of 70–100% and 93–100% respectively (Quigley et al, 2001; Morley et al, 2008). One study, although limited by a small population, provided encouraging results for the use of flow cytometry in cats with IMHA (Limlenglert et al, 2011).
Evaluation of concurrent diseases
The most common pathologies associated with IMHA in the literature include:
- Infectious diseases
- Neoplasia
- Inflammatory diseases
- Drugs
- Toxins
- Vaccines.
Infectious diseases
Although recent evidence suggests that any infection can trigger an immune dysregulation and loss of immune tolerance in predisposed individuals (Rivera-Correa and Rodriguez, 2018), specific microorganisms have been proven to trigger anti-erythrocyte immunoglobulin synthesis.
Haemoparasites such as Babesia gibsoni in dogs (Máthé et al, 2006), and Babesia felis (Schoeman et al, 2001) and Mycoplasma haemofelis in cats (Weingart et al, 2016) have been reported as potential causes of IMHA.
Coombs-positive anaemias have also been observed with Leishmania spp., Dirofilaria immitis and Bartonella spp. in dogs and feline leukaemia virus-infected cats, but evidence showing the production of auto-antibodies in these cases is currently lacking (Garden et al, 2019).
Neoplasia
In humans, IMHA is a recognised paraneoplastic syndrome (Ugoeke et al, 2017; Yu et al, 2017), but this remains unclear in dogs and cats. Veterinary literature is limited to single case reports of dogs with IMHA and concurrent intestinal lymphoma (Prihirunkit and Chootesa, 2016) and leiomyosarcoma (Yuki and Naitoh, 2019), thoracic and splenic sarcoma (Mellanby et al, 2004), acute monoblastic leukaemia (Kreangsak et al, 2010) and seminoma (Wakamatsu and Takenaka, 2000). There is also one report of IMHA in a cat with renal adenocarcinoma (Brown et al, 2019).
Inflammatory diseases
There is evidence that systemic inflammation is a common consequence of IMHA, with free red blood cell fragments acting as potent danger-associated molecular patterns driving the release of inflammatory mediators (Griebsch et al, 2009; Jeffery et al, 2015). Some studies suggest a potential association between pancreatitis and IMHA, either as a trigger factor or as a consequence of systemic cytokine release and thromboembolic events (Guadarrama-Olhovich et al, 2013; Zoia and Drigo, 2017). However, the current supporting evidence remains sparse in both dogs and cats.
Drugs and toxins
Drug-induced IMHA is a rare but recognised occurrence in people (Garratty, 2009). Its pathophysiology remains unclear, but may involve multiple mechanisms, including anti-drug IgG attaching to drug-coated red blood cell membranes and subsequent clearance by the mononuclear phagocytic system (Garratty, 2009).
In veterinary medicine, the evidence supporting the occurrence of drug-induced IMHA is again limited to a handful of case reports potentially associating IMHA with administration of cephalosporin (Bloom et al, 1988), anti-lymphocyte globulin (DeLong et al, 1990), paracetamol (Raguvaran et al, 2019) and propylthiouracil in cats (Peterson et al, 1984; Aucoin et al, 1985).
Toxin exposure is also a reported putative trigger for IMHA. Four cases of IMHA were described in dogs presented after snake envenomation (Ong et al, 2015), although these animals had also received anti-venom and fresh frozen plasma. A dog that presented with honey-bee envenomation later developed haemolytic anaemia with spherocytosis, thrombocytopenia, haemoglobinaemia and haemoglobinuria (Nair et al, 2019).
Vaccines
A causal relationship has been previously inferred, when a close temporal association was found between vaccination and onset of IMHA. One study showed that 26% of dogs diagnosed with IMHA had been vaccinated within 30 days before presentation (Duval and Giger, 1996), but the significance of this remains unclear given the broad practice of vaccination. Another study found no difference in timing of last vaccination and hospital presentation between dogs presenting with IMHA and dogs presenting for other reasons (Carr et al, 2002).
Common concurrent clinicopathological abnormalities
An inflammatory leukogram in the complete blood cell count is a common finding in dogs, with leukocytosis and left shift neutrophilia reported in up to 99% and 80% of animals, respectively (Day, 1996; Reimer et al, 1999; Scott-Moncrieff et al, 2001).
In cats, lymphocytosis has been commonly reported (15–52%) (Husbands et al, 2002; Kohn et al, 2006; Swann et al, 2016).
Several studies also showed 65–70% of dogs with IMHA had concurrent mild thrombocytopenia (<200.000/μl), with 20% having a severely decreased platelet count (< 50.000/μl) (Carr et al, 2002; Orcutt et al, 2010). Similar percentages have been shown for feline patients (Kohn et al, 2006; Swann et al, 2016). The causes of thrombocytopenia in animals with IMHA are likely multifactorial, including immune-mediated destruction, consumption secondary to hypercoagulability and splenic sequestration (Kohn et al, 2006).
According to coagulation testing, 85–100% of dogs are in a hypercoagulable state upon presentation (Sinnott and Otto, 2009; Fenty et al, 2011; Goggs et al, 2012). Interestingly, the reported prevalence of disseminated intravascular coagulation in dogs with IMHA is not as high (28–45%) (Scott-Moncrief et al, 2001; Carr et al, 2002). In cats, one study reported a disseminated intravascular coagulation incidence of 5% altered prothrombin time and activated partial thromboplastin time in 15% of the study population, with no thromboembolic complications observed. However, only 19 cats were recruited for this study (Kohn et al, 2006).
In measurements of serum biochemistry, 60–100% of animals show hyperbilirubinaemia (Kohn et al, 2006; MacNeill et al, 2019) resulting from a combination of increased delivery of bilirubin to the liver and a decreased hepatic clearance, caused by hypoxic hepatocellular damage, which also explains the prevalence of elevated liver enzymes (McCullough, 2003; Kohn et al, 2006).
Azotaemia may also be explained by hypoxia, but renal damage can also occur with persistent saturation of tubular capacity to reabsorb free-haemoglobin (McCullough, 2003). Immunemediated renal impairment has also been reported in dogs with IMHA as well as concurrent distal renal tubular acidosis (Shearer et al, 2009).
Conclusions
IMHA is an important cause of haemolysis in both dogs and cats. An appropriate diagnostic approach aids in differentiating associative (secondary) from non-associative (primary or idiopathic) IMHA, tailoring therapy and identifying poor prognostic indicators.
KEY POINTS
- Immune-mediated haemolytic anaemia is a common cause of anaemia in dogs and, to a lesser extent, cats.
- Primary or non-associative immune-mediated haemolytic anaemia is more common than its secondary/associative counterpart, in both dogs and cats.
- The presence of other conditions needs to be determined, to achieve an appropriate diagnosis of associative or non-associative immune-mediated haemolytic anaemia, as there are no specific tests to differentiate them.
- Neutrophilia in dogs and lymphocytosis in cats are often present, as well as hyperbilirubinaemia with elevated hepatic and renal markers.
- There is no pathognomonic abnormality for the diagnosis of immunemediated haemolytic anaemia.
- A subset of animals with immune-mediated haemolytic anaemia will not present with a highly regenerative anaemia. Consideration should be given that red cell precursors in the bone marrow may be targeted as well.