Epilepsy is a chronic pathological condition of the brain, characterised by recurrent seizures in association with an enduring alteration of the brain (Berendt et al, 2015). The true prevalence of epilepsy in dogs is unknown, but has been estimated to be 0.6–0.75% of the general dog population (Berendt et al, 2015).
Seizures are the clinical manifestation of an excessive, synchronous and uninterrupted discharge of a group of neurons, which causes uncontrollable electrical activity in the cerebral cortex.
Abnormal neuronal activity may be caused by a variety of underlying aetiologies. An extracranial disturbance of metabolic, nutritional or toxic nature might cause reactive seizures in a normal brain, but structural epilepsy is caused by intracranial lesions affecting the brain, which can initially be caused by infections, inflammations, neoplasia, vascular accidents and trauma. No definitive causes have yet been found in dogs suffering from benign idiopathic epilepsy, although a genetic component may be involved. However, if a structural cause is suspected, despite remaining obscure, the disease is defined as unknown epilepsy (Berendt et al, 2015). A study among dogs undergoing magnetic resonance imaging (MRI) for investigation of epilepsy reported the presence of structural lesions in 45% of dogs, and a presumed diagnosis of idiopathic epilepsy in 53.8% of dogs (Hall et al, 2020).
In humans, a response to anti-epileptic medication is considered adequate when there is a reduction in seizure frequency of at least 50% (Regesta and Tanganelli, 1999). In veterinary medicine, the term refractory or pharmacoresistant epilepsy is used for animals experiencing side effects, or inadequate seizure control, despite appropriate treatment (Thomas, 2000). However, before concluding that an animal is not responding to treatment, it is important to investigate any errors such as the use of incorrect drugs, short duration of treatment, inappropriate dosage or poor owner compliance (Thomas, 2000).
Pharmacoresistant epilepsy is defined as failure to achieve freedom from seizures despite adequate administration of two (or more) well-tolerated and correctly chosen anti-epileptic drugs, whether they have been administered as consequent mono-therapies or in combination (De Risio and Platt, 2014).
Indications for immediate anti-epileptic treatment are:
- Two or more episodes within 6 months (Bhatti et al, 2015)
- Status epilepticus or cluster seizures (Bhatti et al, 2015)
- Postictal period with severe signs or prolonged duration of more than 24 hours (Bhatti et al, 2015)
- Increase in seizure frequency, severity or duration over three interictal periods (Bhatti et al, 2015)
- Presence of structural lesion, or history of brain disease or head trauma (Podell et al, 2016)
The ambition of long-term anti-epileptic treatment is seizure eradication. However, treatment is considered effective when a decrease in seizure frequency, duration and severity above 50% is achieved with the least possible side effects (De Risio and Platt, 2014).
The first choice of medication for long-term treatment of epilepsy is phenobarbital (Kluger et al, 2009; Meland et al, 2019) (Figure 1). The medication is easily available in several formulations and multiple studies support its efficacy compared to other anti-epileptic drugs (Boothe et al, 2012; Stabile et al, 2019).
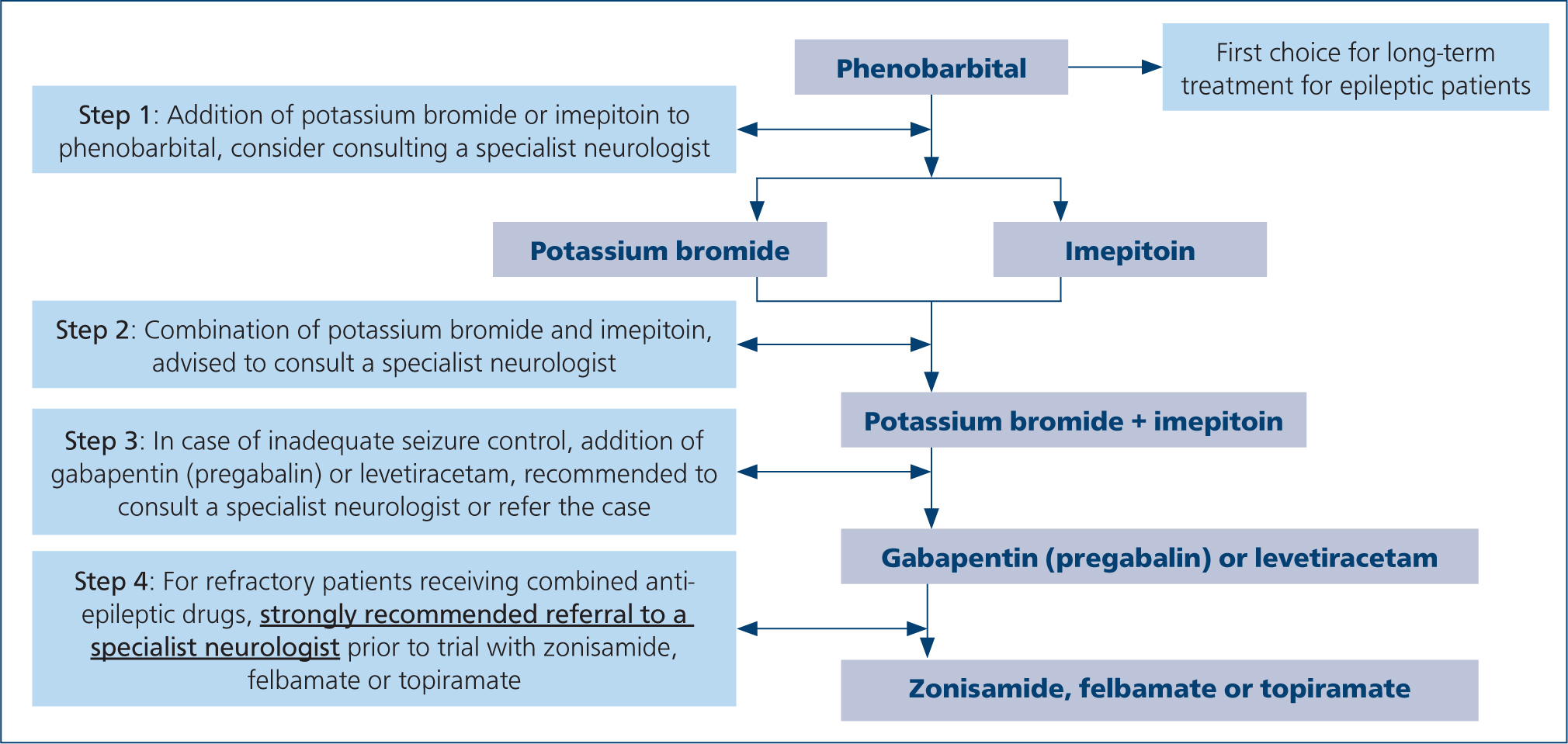
There are cases in which administration of phenobarbital is contraindicated. There are well documented cases of worsening of liver function in patients already suffering from hepatic disease, as well as idiosyncratic hepatotoxicity, caused by phenobarbital (Bunch et al, 1982; Dayrell-Hart et al, 1991). Concurrent administration of phenobarbital and other potentially hepatotoxic medications can increase the risk of hepatotoxicity and should be avoided (Dewey and Da Costa, 2016). Regarding side effects, phenobarbital usually causes mild and transient reactions including sedation, ataxia, polyphagia, polyuria and polydipsia, although in some cases, these side effects may lead to a poor quality of life (Chang et al, 2006; De Risio and Platt, 2014). Additionally, transient hyperexcitability, hyperactivity, restlessness and aggression have been occasionally associated with phenobarbital administration (Farnbach, 1984; Chang et al, 2006). Blood and bone marrow dyscrasia, superficial necrolytic dermatitis, pancreatitis and dyskinesia have also been reported in dogs treated with this medication (Gaskill and Cribb, 2000; March et al, 2004; Kube et al, 2006; Haböck and Pakozdy, 2012; Bersan et al, 2014) (Figure 2). If the side effects are not tolerable, the suggestion is to gradually withdraw the phenobarbital and to start an alternative drug. A suggested protocol is to reduce the dose by 25% each month (Penderis and Volk, 2013). A second drug may be needed in adjunction, if phenobarbitol alone is not enough to reduce the seizure frequency and/or severity (De Risio and Platt, 2014).
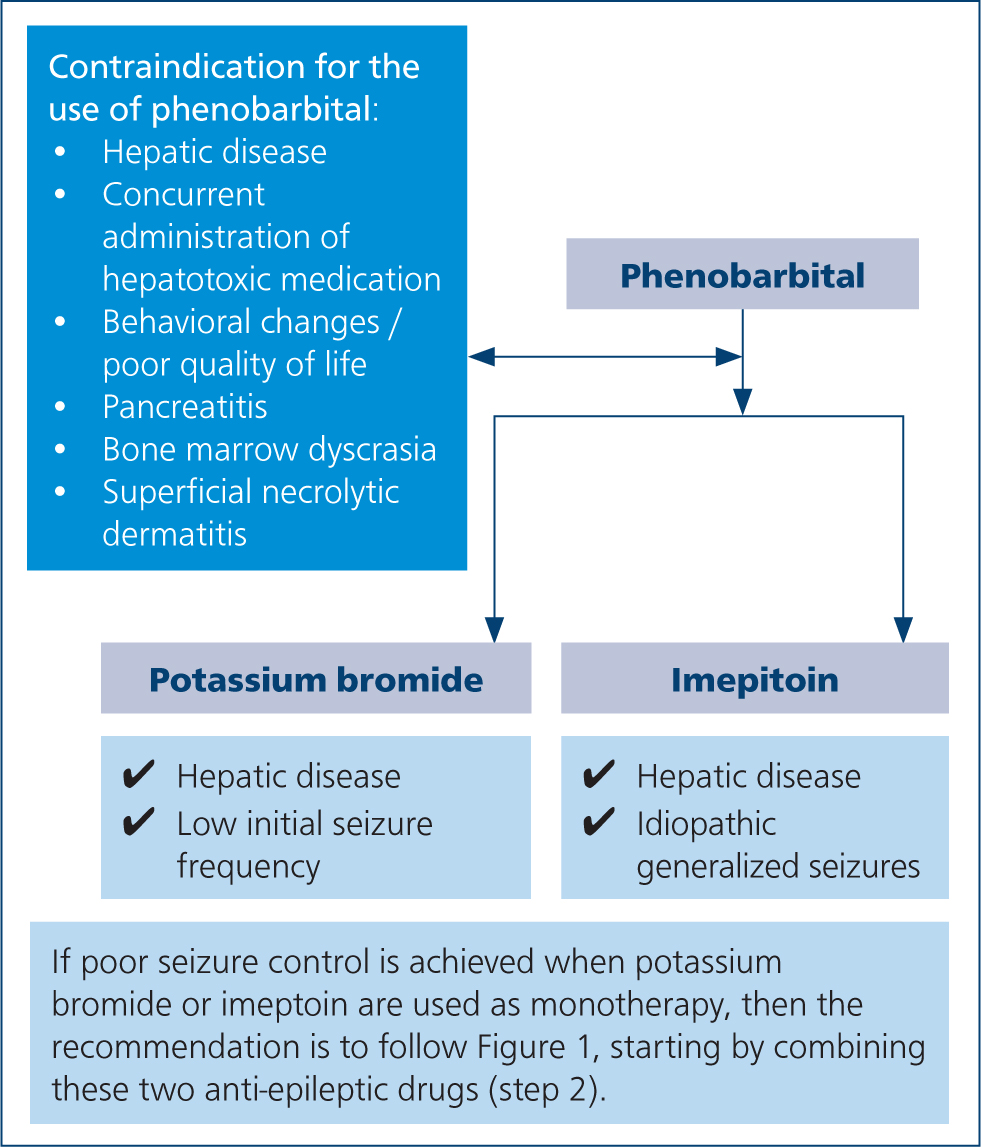
The most common side effects of phenobarbital are mild and usually transient, and include sedation, ataxia, polyphagia, polyuria and polydipsia (De Risio and Platt, 2014).
Furthermore, phenobarbital undergoes hepatic metabolism and it is contraindicated in animals with impaired hepatic functions. It should also not be used to control seizures resulting from hepatic disease causing hepatic encephalopathy (De Risio and Platt, 2014).
Phenobarbital has been reported to cause both chronic dose dependent hepatic cirrhosis and acute idiosyncratic hepatic failure (Bunch et al, 1982; Dayrell-Hart et al, 1991). Concurrent administration of phenobarbital and other potentially hepatotoxic medications can increase the risk of hepatotoxicity and should be avoided.
Cytopenia, superficial necrolytic dermatitis and pancreatitis have been rarely reported in dogs (De Risio and Platt, 2014). If the side effects are not tolerable, the suggestion is to quickly withdraw the medication and to start an alternative drug. A second drug may be needed in adjunction if phenobarbital alone is not enough to handle the seizure frequency (De Risio and Platt, 2014).
This article summarises guidelines regarding the various alternatives for anti-epileptic treatment, taking into consideration specific characteristics of each anti-epileptic drug, to help clinicians decide which anti-epileptic drug should be started, added or changed to.
Alternatives used for monotherapy or first-line adjunctive anti-epileptic drugs
Bromide
Potassium bromide, often referred to as bromide, is an inorganic halide, recommended as add-on anti-epileptic drug in dogs with epilepsy resistant to first-line drug therapy (Charalambous et al, 2014) (Figure 1). A study concluded the superiority of phenobarbital over potassium bromide, when used as monotherapy in dogs with idiopathic epilepsy, when the animals receiving phenobarbital demonstrated a significantly greater percentage of seizure eradication or reduction of seizure duration, as well as a lower incidence of long-term adverse effects (Boothe et al, 2012). However, potassium bromide as an adjunctive therapy has been shown to reduce successfully the seizure frequency in a high percentage of dogs resistant to phenobarbital monotherapy, even decreasing severity and intensity of seizures (Podell and Fenner, 1993; Trepanier et al, 1998). Regarding its efficacy as adjunctive antiepileptic drug, conflicting results are reported in the literature (Charalambous et al, 2014); however, the drug is commonly used as the first add-on to dogs resistant to phenobarbital (Podell and Fenner, 1993; Trepanier et al, 1998; Chang et al, 2006).
Bromide may represent the first choice anti-epileptic in dogs with hepatic dysfunction or those with concurrent disorders requiring therapy that affects the liver, for which treatment with phenobarbital is contraindicated (De Risio and Platt, 2014) (Figure 2).
The mechanism of action of bromide is not completely understood, but seems to involve gamma-aminobutyric acid (GABA) receptor gated ion channels (Suzuki et al, 1994). The GABA receptor is the major inhibitory neurotransmitter of the brain. Bromide ions of a small diameter compete with the larger chloride ions and, as a consequence, cross more easily through neuronal channels; meaning only two chloride ions enter the GABA receptors for every three bromide ions that enter (Figure 3). This results in post-synaptic hyperpolarization and reduced neuronal excitability (Pearce, 1990; Podell and Fenner, 1993; Trepanier and Babish, 1995; Lugassy and Nelson, 2009).
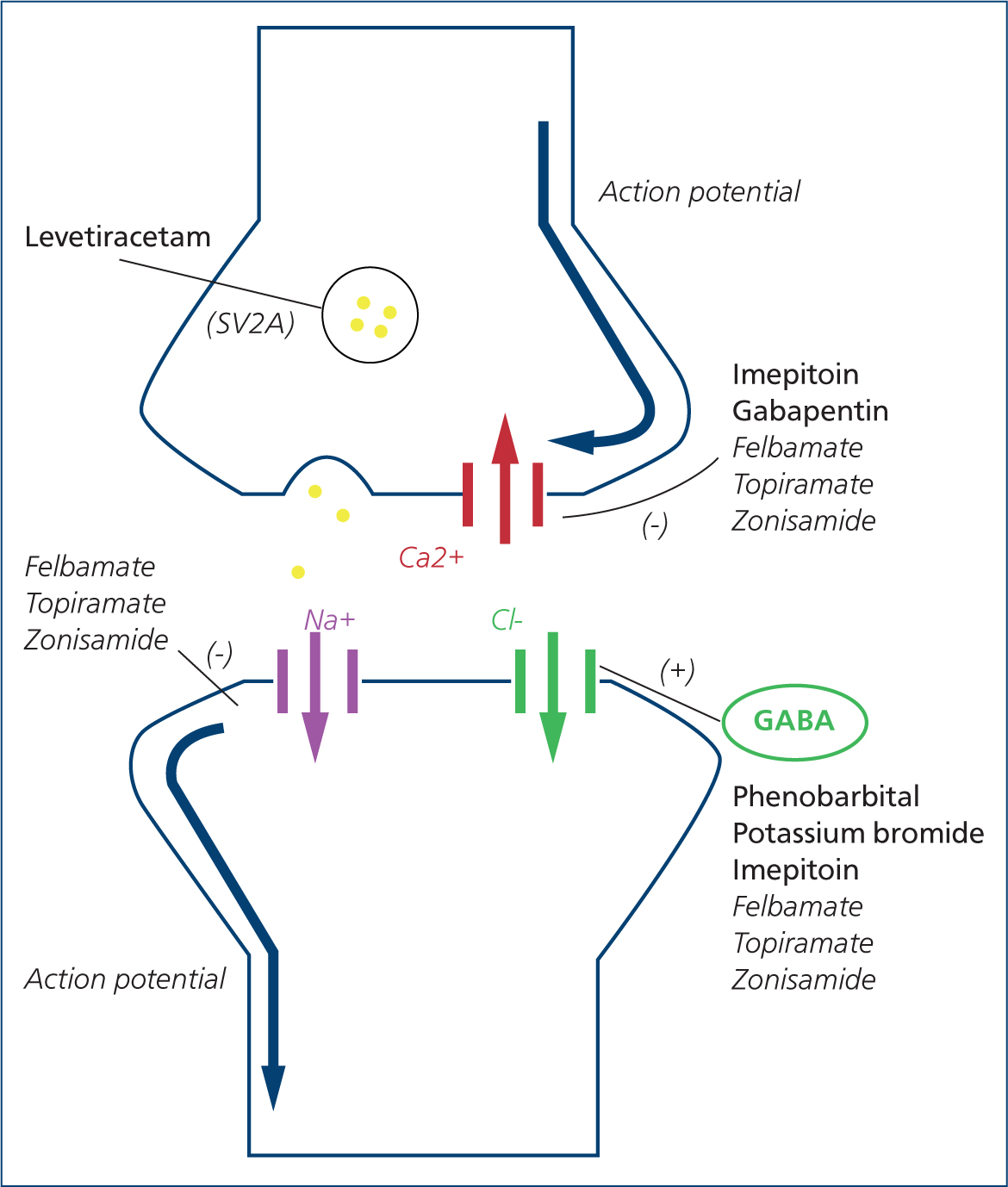
Considering bromide's pharmacokinetics, the bioavailability reaches approximately 50%, and it takes approximately 3 months for the drug to reach the steady state concentration after the start of maintenance therapy. Serum reference ranges can be monitored 1–3 months after treatment initiation and 1 month after dose adjustment (Podell et al, 2016). Reference serum bromide concentrations are, 2000–3000 mg/litre when bromide is used as monotherapy, and 1000–2000 mg/litre when bromide is used in combination with phenobarbital (Dowling, 1994). Bromide is not metabolised in the liver and is excreted unchanged in the urine (Trepanier and Babish, 1995).
After glomerular filtration, bromide ions are reabsorbed by the renal tubules in competition with chloride ions. This is why high chloride intake with food increases the excretion and shortens the half-life of bromide and vice versa, making dietary changes in patients receiving such treatment undesirable (Trepanier and Babish, 1995; Shaw et al, 1996). Moreover, the use of loop diuretics such as furosemide may enhance bromide elimination and lower serum concentrations (Millns and Rogers, 1978).
Pseudohyperchloraemia may be detected on biochemistry profiles, because of the similarity between chloride and bromide ions (Wenk et al, 1976; De Risio and Platt, 2014).
The recommended initial dose for bromide monotherapy in dogs is 30–40 mg/kg as one or two doses daily, or 20–30 mg/kg as one or two doses daily when administered as an adjunctive therapy. A loading dose protocol for bromide can be used when rapid achievement of high concentrations is required. This can be achieved by administering 600 mg/kg total dose, together with 30 mg/kg/day maintenance dose, in divided doses over a 48-hour period (Table 1). This protocol is often associated with adverse effects, thus hospitalisation is recommended. Serum bromide concentration should be checked after the end of the loading dose, to provide an indicative value to be considered along with the clinical response (Gindiciosi et al, 2014).
Table 1. Indications and recommended doses for each anti-epileptic drug for canine epileptic patients when phenobarbital is not enough or not indicated
Anti-epileptic drug | Indications | Dose |
---|---|---|
Potassium bromide (Monotherapy or 1st or 2nd line adjunctive) |
|
|
Imepitoin (Monotherapy or 1st or 2nd line adjunctive) |
|
|
Levetiracetam (2nd or 3rd line adjunctive) |
|
|
Zonisamide (3rd, 4th or 5th line anti-epileptic drug) |
|
|
Gabapentin and pregabalin (3rd, 4th or 5th line anti-epileptic drug) |
|
|
Felbamate and topiramate (3rd, 4th or 5th line anti-epileptic drug) |
|
|
The common, usually transient and dose-dependent, adverse effects of potassium bromide are sedation, ataxia, pelvic limb weakness, polydipsia, polyuria and polyphagia. Gastrointestinal signs such as pancreatitis, vomiting and diarrhoea have also been reported (Pearce, 1990; Podell and Fenner, 1993; Trepanier and Babish, 1995; Chang et al, 2006; Steiner et al, 2008) (Table 2).
Table 2. Common adverse effects of each anti-epileptic drug, divided into four categories
Type I: predictable and dose-dependent | Type II: idiosyncratic and potentially life threatening | Type III: cumulative with long-term treatment and potentially life threatening | Type IV: delayed (carcinogenic or teratogenic) and life-threatening | |
---|---|---|---|---|
Bromide |
|
|
|
- |
Imepitoin |
|
- | - |
|
Levetiracetam |
|
|
|
- |
Zonisamide |
|
|
- | - |
Gabapentin |
|
- | - | - |
Felbamate |
|
|
- | - |
Topiramate |
|
- | - | - |
Excessive sedation and nausea often respond to dose division every 12 hours, and administration of liquid solution instead of tablets or capsules (Trepanier and Babish, 1995; Baird-Heinz et al, 2012). Erythematous dermatitis and nodular/pustular skin lesions have been rarely reported in dogs treated with bromide and are known as bromoderma. Bromism, or bromide toxicity, is a syndrome associated with serum bromide concentrations towards the upper end of the reference range, with a variety of clinical signs of peripheral and central nervous system dysfunction. This includes obtundation, stupor or coma, bilateral mydriasis with slow and incomplete pupillary light reflexes, bilateral blindness, anisocoria, abnormal behaviour, head pressing, ataxia, paraparesis, tetraparesis with normal or decreased spinal reflexes, dysphagia, megaesophagus and muscle pain (Baird-Heinz et al, 2012) (Table 2). Decreasing bromide dose by 25–50% is necessary in such cases and is usually enough to reduce symptoms. In some cases, administration of 0.9% sodium chloride is advised in order to enhance renal excretion of bromide and achieve a faster resolution of symptoms of toxicity (Rossmeis and Inzana, 2009).
Imepitoin
Imepitoin is a next generation anti-epileptic drug with anticonvulsant and anxiolytic properties. It was licensed in 2013 for use in idiopathic epileptic dogs with generalised seizures (Rundfeldt et al, 2014) (Figure 2).
Imepitoin mainly acts by binding at the benzodiazepine binding site of GABA receptors, resulting in neuronal hyperpolarization (Sigel et al, 1998). In addition, imepitoin has a mild blocking effect on voltage-activated calcium channels (Bialer et al, 1999). Its anxiolytic effect is believed to be caused by antagonising the corticotrophin-releasing factor in the level of brainstem neurons (Rostock et al, 1998). The results of studies on its anxiolytic effect are controversial, with two studies concluding that there is insufficient evidence to support it (McPeake and Mills, 2017; Packer et al, 2017), whereas another study had encouraging results regarding the use of imepitoin for canine anxiety control (Engel et al, 2019).
The pharmacokinetic profile of imepitoin is advantageous. It is well absorbed after oral administration and rapidly crosses the blood–brain barrier to reach a steady state from the first dose. Fasting is recommended, since the bioavailability of imepitoin is higher when administered in fasted animals. Imepitoin is extensively metabolised in the liver and excreted mainly via the faecal route. The limited renal metabolism and urinary excretion is beneficial in animals with renal impairment (De Risio and Platt, 2014).
Imepitoin is only available as an oral formulation. A study comparing oral and rectal administration of imepitoin in healthy dogs demonstrated significantly lower plasma concentrations of the drug when given rectally (Martlé et al, 2020). The recommended starting dose is 10mg/kg every 12 hours, which can be increased weekly, depending on response, up to 30mg/kg every 12 hours (Table 1). Imepitoin has not been associated with tolerance or dependence issues during long-term administration (Bhatti et al, 2015).
The most common adverse reactions reported are mild and commonly transient, seen at the beginning of treatment (Löscher et al, 2004; Rieck et al, 2006). They include polyphagia, hyperactivity, polyuria, polydipsia, hypersalivation, ataxia, emesis, lethargy, diarrhoea, prolapsed nictitating membranes, decreased vision and sensitivity to sound (Bhatti et al, 2015) (Table 2). Overall, imepitoin is considered a safe medication, with a low potential for toxicity even in high doses. In cases of intoxication, symptoms may arise from the central nervous system or the gastrointestinal tract and usually respond to symptomatic treatment. Signs of aggression or ataxia have been reported to resolve after discontinuation of the medication (Stabile et al, 2019). Therapeutic monitoring of serum imepitoin concentration is not advised, since it is a medication with a wide therapeutic index and no established serum reference range for dogs (Löscher et al, 2004).
The fact that imepitoin partially antagonises the benzodiazepine binding site of the GABA receptor does not preclude the use or efficacy of diazepam for status epilepticus in epileptic dogs on long term treatment with this medication. However, in these cases, an additional anti-epileptic drug to diazepam might be required parenterally (such as phenobarbital or levetiracetam). In terms of its anxiolytic effect, two studies were performed in 2017. Imepitoin was found to help reduce stress-related problems in healthy dogs, but no significant improvement was seen in epileptic dogs with anxiety issues (McPeake and Mills, 2017; Packer et al, 2017).
Several studies have investigated the efficacy of imepitoin in dogs affected by idiopathic epilepsy. In one study, imepitoin was found to be effective for dogs with recently diagnosed epilepsy, unless they had a history of cluster seizures which could not be controlled using imepitoin (Rundfeldt et al, 2015). Comparison of phenobarbital to imepitoin as monotherapy in idiopathic epileptic patients has shown various results, most of which suggest a better effectiveness of phenobarbital over imepitoin (Löscher et al, 2004; Stabile et al, 2019). However, one study found a similar decrease in seizure frequency in dogs treated with phenobarbital or imepitoin as monotherapy (Tipold et al, 2015). The addition of imepitoin at a low starting dose of 5mg/kg every 12 hours to the treatment of idiopathic epileptic dogs, refractory to one or more anti-epileptic drugs, was well tolerated and resulted in a reduction of monthly seizure frequency (Neßler et al, 2016). Moreover, idiopathic epileptic dogs resistant to the maximum dose of imepitoin responded well to the addition of phenobarbital or potassium bromide to the treatment protocol (Royaux et al, 2017). The gradual withdrawal of imepitoin from these animals was not found to increase seizure frequency. On the contrary, the owners reported good seizure control and a decrease in treatment-related side effects. A 3-month tapering period was used, safely reducing the dose of imepitoin by 50% monthly (Stee et al, 2017).
A systematic review on the evidence for the use of imepitoin concluded that there is sufficient evidence for its effectiveness when used as monotherapy, but insufficient evidence for its use as adjunctive treatment (Charalambous et al, 2014). More recent studies demonstrate its use as adjunctive treatment to be safe and effective (Neßler et al, 2016; Royaux et al, 2017) (Figure 1).
Adjunctive anti-epileptic drugs for refractory epileptic canine patients
Levetiracetam
Levetiracetam is a new anti-epileptic drug structurally related to piracetam, with antinociceptive and neuroprotective properties (Rossetti et al, 2014). It is licensed as monotherapy and for the adjunctive treatment of focal or generalised seizures (Berkovic et al, 2007; Noachtar et al, 2008). Levetiracetam is indicated in cases of poor seizure control with first-line anti-epileptic drugs in cases of idiopathic epilepsy with a tendency for cluster or status epilepticus; in animals with advanced hepatic disease, or as a monotherapy in dogs with structural epilepsy (Volk et al, 2008; De Risio and Platt, 2014; Packer et al, 2015). Regarding its use as a monotherapy, two studies conclude that it can be an option for dogs with structural epilepsy (Kelly et al, 2017), but its efficacy is overall poor in animals with idiopathic epilepsy (Fredsø et al, 2016). Levetiracetam has been described as preventive therapy for animals undergoing surgery for portosystemic shunt, to reduce the risk of postoperative seizures (Fryer et al, 2011).
The mechanism of action is not fully understood, but it is distinct from other anti-epileptic drugs. Levetiracetam binds selectively to the integral membrane synaptic vesicle protein 2A on the presynaptic terminal. This results in altered synaptic vesicle fusion and altered release of neurotransmitters (De Risio and Platt, 2014). Other suggested mechanisms of action, more similar to those of other drugs, include the modulation of current through voltage-gated calcium channels, the inhibition of glutamate release, the intervention in GABA and glycine-gated currents and the antagonism of the burst firing of neurons (Dewey, 2006; Muñana, 2013; De Risio and Platt, 2014) (Figure 3).
In terms of pharmacokinetics, levetiracetam resembles an ‘ideal’ anti-epileptic drug (Patsalos, 2000). It is rapidly absorbed when administered orally, parenterally or rectally, with a bioavailability of almost 100% and a peak concentration reached within 1 hour post-oral administration. The absorption is delayed but not decreased when administered with food. The basic sites of metabolism are the kidneys, and the drug is excreted mainly unchanged in the urine. Levetiracetam does not undergo hepatic metabolism via the cytochrome P450 (Moore et al, 2011).
Levetiracetam can be administered orally, intravenously, intramuscularly and subcutaneously, at a recommended initial dose of 20 mg/kg every 8 hours (owing to the short half-life of 3–4 hours) (Table 1). One study reports no tissue damage post-extravasation and good tolerability, without significant signs of pain during intramuscular injection (Patterson et al, 2008).
The development of tolerance, also called ‘honeymoon effect’, has been described for dogs treated with levetiracetam as an adjunctive therapy to phenobarbital and bromide (Volk et al, 2008). Because of concerns about the development of tolerance, as well the high cost of levetiracetam, a pulse treatment protocol has been suggested (Volk et al, 2008; Packer et al, 2015). The protocol consists of the administration of 60 mg/kg after a seizure occurs, followed by 20 mg/kg every 8 hours until a seizure-free period of 48 hours is reached, at which point levetiracetam administration is ceased (Table 1). Pulse treatment can be valuable in patients with a tendency for cluster seizures or status epilepticus. The protocol can be repeated whenever a seizure occurs, or when the owner recognises signs of an upcoming episode (Volk et al, 2008; Packer et al, 2015).
The dose of levetiracetam may require adjustment when given concurrently with phenobarbital. In fact, in this case an increased clearance of levetiracetam has been demonstrated, leading to decreased serum concentrations and a shorter half-life (Moore et al, 2011).
Levetiracetam is a safe medication with only a few mild reported side effects and low potential for toxicity, even in high doses. In dogs, the most common side effects are sedation, ataxia, restlessness, decreased appetite and vomiting (Volk et al, 2008; Muñana et al, 2012) (Table 2). Given the safety of the medication and the lack of an established serum reference range for dogs and cats, monitoring of serum levetiracetam concentration is not generally performed and it is advised mainly in order to individualise treatment (Volk et al, 2008). Although considered a safe medication, a study indicates the need for dose adjustment in animals suffering from chronic kidney disease, given that the kidneys are the main site of metabolism (So-Yeon, 2020). One case report describes a fatal adverse reaction, post-rapid intravenous injection of undiluted levetiracetam at 60 mg/kg. That dog developed tachycardia, hyperglycaemia, hypotension, a dull mentation and respiratory failure caused by pulmonary oedema, leading to cardiac arrest (Biddick et al, 2018). Behavioural changes, both positive (such as increased activity and a calmer mood) and negative (such as anxiety and aimless behavior), have also been described in dogs undergoing treatment with levetiracetam as monotherapy, add-on treatment or pulse therapy (Erath et al, 2020).
Overall, levetiracetam appears to be a safe medication for canine patients, with a better efficacy when used as pulse treatment compared to long-term administration, and is recommended as adjunctive therapy for canine epileptic patients (Charalambous et al, 2014; Packer et al, 2015) (Figure 1).
Zonisamide
Zonisamide is a sulphonamide-based antiepileptic drug licensed for use in epileptic dogs in Japan and available in the UK (Bhatti et al, 2015).
Zonisamide is believed to act via various mechanisms of action, such as blockage of voltage-gated sodium and calcium channels, enhancement of GABA release, inhibition of glutamate release, neuronal membrane stabilisation and protection and facilitation of dopaminergic and serotonergic transmission (Leppik, 2004; Biton, 2007) (Figure 3).
Zonisamide is well absorbed after oral administration, and has a relatively long elimination half-life and a low level of protein binding. The drug undergoes hepatic metabolism and is then excreted by the kidneys. Coadministration with phenobarbital increases zonisamide clearance and alters its pharmacokinetics. For this reason, the recommended initial dose of 3–7 mg/kg orally every 12 hours can rise up to 7–10 mg/kg orally every 12 hours when administered as adjunctive to phenobarbital treatment (Dewey et al, 2004; Boothe and Perkins, 2008) (Table 1).
Reported zonisamide adverse effects in dogs are usually mild and transient and include sedation, ataxia, vomiting and inappetence (Dewey et al, 2004; Von Klopmann et al, 2007; Chung et al, 2012). Nonetheless, idiosyncratic reactions have been described, including allergic reactions to sulfonamide-containing medications, keratoconjunctivitis sicca, polyarthropathy, acute toxic hepatopathy and renal tubular acidosis (Dewey et al, 2004; Cook et al, 2011; Schwartz et al, 2011) (Table 2).
Preliminary results on zonisamide efficacy as monotherapy and as an adjunctive anti-epileptic medication in epileptic dogs seem to be encouraging (Dewey et al, 2004; Von Klopmann et al, 2007; Chung et al, 2012). However, there is not enough evidence to recommend its use in either case, and larger studies are warranted (Charalambous et al, 2014) (Figure 1). At this stage, the authors would recommend the use of zonisamide as an add-on treatment for refractory epileptic animals with no history of hepatic or renal disease.
Gabapentin and pregabalin
Gabapentin and pregabalin have a similar structure and mechanism of action. To the authors' knowledge, there is very little evidence on the use of pregabalin for the treatment of seizures in canine patients (Dewey et al, 2009), and there is limited available data regarding pharmacokinetics, effective doses and adverse reactions, so pregabalin is not described in detail in this report.
The anti-epileptic effect of gabapentin is believed to be caused by blockade of voltage-gated calcium channels (Field et al, 2006) (Figure 3). Gabapentin is also efficient for neuropathic and postoperative pain. It has been licensed in humans for the adjunctive treatment for focal seizures, with or without secondary generalisation, and for post-herpetic neuralgia (Bockbrader et al, 2010).
Gabapentin is well absorbed after oral administration and peak plasma concentrations are reached within 1–3 hours (Radulovic et al, 1995). It is mainly excreted by the kidneys without inducing hepatic cytochrome P450, with an elimination half-life of approximately 3 hours (Bhatti et al, 2015). The main reported side effects in dogs are sedation and ataxia (Govendir et al, 2005).
The recommended starting dose for gabapentin is 10–20 mg/kg orally every 6–8 hours (De Risio and Platt, 2014) (Table 1). The dose may require reduction in animals with impaired renal function (Bockbrader et al, 2010). Withdrawal symptoms such as mental status changes, anxiety and rarely, cases of status epilepticus have been described in humans after sudden drug discontinuation, thus tapering the dose over at least 1 week is recommended (Norton, 2001).
Regarding its efficacy as an adjunctive treatment to phenobarbital or bromide, the available data do not support a significant success in seizure frequency reduction using gabapentin (Charalambous et al, 2014). To the authors' knowledge, the effectiveness of gabapentin as a monotherapy for treatment of canine epileptic seizures has not been studied.
Felbamate and topiramate
There is limited information regarding the efficacy of these anti-epileptic drugs in dogs (Bhatti et al, 2015).
Felbamate's mechanism of action is based on inhibition of intracellular calcium currents and blockade of voltage-gated sodium channels (White, 1999) (Figure 3). The drug is almost entirely absorbed after oral administration, reaching the peak concentration between 2 and 6 hours. Felbamate is partially metabolised by the liver and is excreted by the kidneys, and has a short elimination half-life (Adusumalli et al, 1992). Felbamate increases phenobarbital serum levels in a dose-dependent manner (Bourgeois, 1997), and its elimination was reported to be largely reduced when given concurrently with gabapentin (Hussein et al, 1996). The recommended starting dose is 20 mg/kg orally three times daily, which can be increased up to 70 mg/kg three times daily depending on the response (Adusumalli et al, 1992) (Table 1). To the authors' knowledge, studies recommending a safe upper limit dose in dogs are missing. The main side effects reported in dogs are keratitis sicca and haematological abnormalities such as thrombocytopenia and leucopoenia, resulting from bone marrow suppression (Ruehlmann et al, 2001; Dewey, 2006). Additionally, hepatic dysfunction has been identified in animals undergoing treatment with felbamate and phenobarbital, although which drug is the origin of liver damage remains uncertain (Dayrell-Hart et al, 1991) (Table 2).
Only one study has determined the use of felbamate to be successful in controlling focal seizure activity in dogs (Ruehlmann et al, 2001).
Topiramate is believed to act by potentiating the activity of GABA and inhibiting voltage-sensitive calcium and sodium channels (Caldwell et al, 2005) (Figure 3). The drug has a short half-life of less than 4 hours after oral administration (Streeter et al, 1995). Almost 80% is excreted unchanged in the urine, so a dose reduction of 50% is advised for animals with renal disease (Lyseng-Williamson and Yang, 2007). Topiramate undergoes significant biliary excretion in the dog (Caldwell et al, 2005).
Topiramate has a low potential for interactions with other anti-epileptic drugs (Bialer et al, 2004). It is not an inhibitor of cytochrome P450 isoenzymes (De Risio and Platt, 2014) and, unlike felbamate, it is believed to have a synergistic action when used with phenobarbital (Czuczwar and Przesmycki, 2001).
Sedation, ataxia and weight loss are the most common side effects in dogs (Shorvon, 1996; Kiviranta et al, 2013) (Table 2). Dosages of 2–10 mg/kg two to three times daily have been described (Kiviranta et al, 2013) (Table 1).
Given the limited evidence regarding their use as anticonvulsant medications in dogs, felbamate and topiramate should be reserved for animals refractory to the other more thoroughly investigated and safer anti-epileptic drugs in this species. The authors do not recommend the use of felbamate and topiramate in a first opinion practice (Charalambous et al, 2014; Bhatti et al, 2015) (Figure 1).
Further considerations for the general practitioner
Despite several anti-epileptic medications being available for canine patients, around 20–30% of dogs with epilepsy will remain poorly managed (Podell and Fenner, 1993; Trepanier et al, 1998). More recently, non-medical treatment options are being explored as an adjunct for those patients (De Risio and Platt, 2014). One option is the use of a specific diet mimicking the human ketogenic diet, which is enriched in medium chain triglyceride oil. A medium chain triglyceride diet has shown some positive results on seizure control and behaviour in dogs with idiopathic epilepsy (Law et al, 2015; Molina et al, 2020; Berk et al, 2021). Nonetheless, more standardised studies on larger samples need to be performed in order to reach conclusions regarding the efficacy and possible side effects of a medium chain triglyceride diet. Another area of current research is the use of cannabidiol as adjunctive treatment of epileptic dogs, but similarly to a medium chain triglyceride diet, there is not enough evidence to support advising its use in veterinary practice (McGrath et al, 2019).
It is the authors' opinion that consultation and referral for advanced investigations should be considered when initial treatment with first or second line anti-epileptic drugs does not ensure a good quality of life for the animal.
Conclusions
Several drugs have been studied as possible anti-epileptic medications. Further studies are warranted to confirm their efficacy as alternatives or adjunctives in dogs affected by epilepsy. Moreover, we need to keep in mind that each case requires a unique approach. Different cases must be carefully evaluated and an individualised anti-epileptic protocol should be formed in order to achieve the desired reduction in seizure frequency and severity.
KEY POINTS:
- Potassium bromide and imepitoin can be used as alternative monotherapies to phenobarbital and are the first line adjunctive anti-epileptic drugs.
- Gabapentin, zonisamide and levetiracetam can be added to the treatment protocol of animals with persistent seizures despite treatment with one or more first line anti-epileptic drugs.
- Levetiracetam is a safe and well tolerated anti-epileptic drug, suitable for use as an adjunctive treatment in poorly controlled epileptic animals, with the advantageous option of use in pulse therapy when a rapid result is required.
- Felbamate, topiramate, medium chain triglyceride diet and cannabidiol are options to be considered in case of pharmacoresistant epilepsy, although further studies are required regarding their efficacy
- In cases with incomplete investigations and poor response to treatment, advice from a referral hospital should be sought.